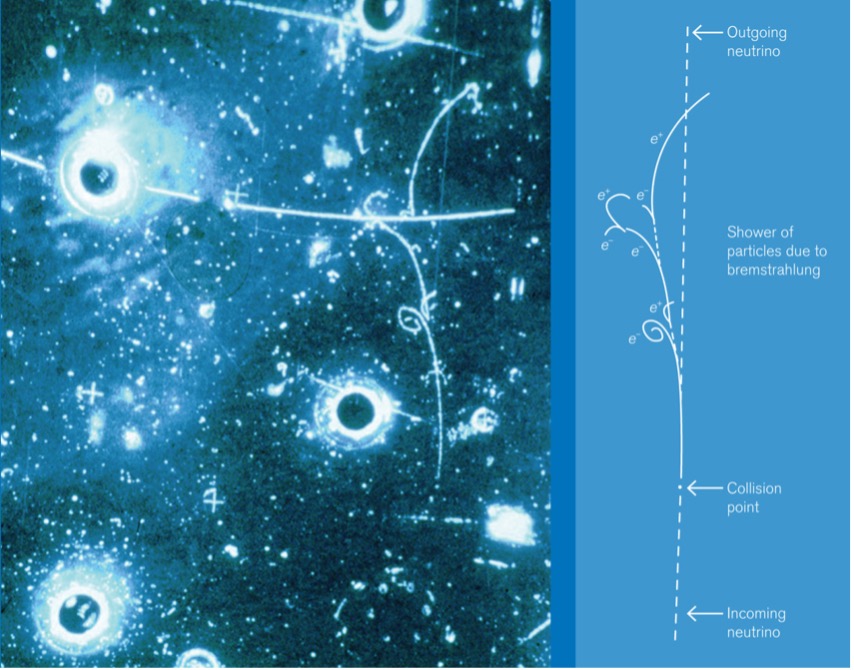
The first leptonic neutral current event spotted with Gargamelle. It shows an incoming neutrino (not visible) arriving from below where it then interacts with an electron (visible track). The interaction radiates photons and then produces electron-positron pairs. Credit: CERN-EX-60100-1/ Kurt Riesselmann
Half a century ago, a series of tiny tracks in a bubble chamber at CERN changed the course of particle physics. The observation of "weak neutral currents", announced on 19 July 1973 by Paul Musset of the Gargamelle collaboration, suggested that the electromagnetic and weak forces are facets of a more fundamental electroweak interaction that ruled in the early Universe. Exploring this new sector of nature has been a core business of CERN ever since, leading to the discovery of the W and Z bosons in 1983 and culminating with the discovery of the Higgs boson in 2012.
The weak force is one of the four fundamental forces of nature, responsible for crucial processes such as radioactive beta decay. Whereas the electromagnetic force was well understood as the result of neutral photons being exchanged between charged particles, the weak interaction was harder to cast in the language of quantum theory. In the 1960s, theorists posited that the weak interaction was mediated by massive versions of the photon: the charged W boson and the neutral Z boson, both inextricably tied up with the photon of electromagnetism. The W boson enabled weak interactions that involved a rearrangement of electrical charge, while the Z boson was how uncharged particles interacted via the weak force. While the former were already known to occur, the latter had never been seen before.
As physicists mastered the art of firing intense beams of neutrinos into detectors to study fundamental interactions, searches for neutral currents became possible. By the late 1960s André Lagarrigue of LAL Orsay had proposed the world's biggest bubble chamber, Gargamelle, named after a fictional giantess. The chamber was built by the École Polytechnique Paris in 1968 and assembled at one of the beamlines of CERN's Proton Synchrotron. Data taking started in 1970, with first results coming in shortly after. Reflecting the focus of experimentalists at the time, the search for neutral currents was placed only eighth in Gargamelle's top-ten physics goals.
Picking out experimental evidence for neutral currents from among numerous similar-looking events was not easy, especially with the technology of the time. Researchers needed to see both "leptonic" events (whereby a neutrino interacted with an electron in the dense gas Gargamelle was filled with) and "hadronic" events (whereby a neutrino was scattered from a proton or neutron). "I remember spending the evenings with my colleagues scanning the films on special projectors, which allowed us to observe the eight views of the chamber," recalls Gargamelle member Donatella Cavalli from the University of Milan, who was a PhD student at the time. "When the first leptonic event was found in December 1972, we were convinced that neutral currents existed."
Further data would reveal candidate hadronic neutral-current events, but it took time for the community to be convinced. Initially, the independent Harvard-Pennsylvania-Wisconsin-Fermilab experiment in the US confirmed Gargamelle's findings, but when they changed their experimental set-up, the tracks vanished. Only in 1974, after further analysis by both collaborations, was the existence of neutral currents universally accepted - leading to the award of the 1979 Nobel Prize in Physics to electroweak architects Sheldon Glashow, Abdus Salam and Steven Weinberg.
Gargamelle is now an exhibit in CERN's Van Hove Square, but physicists are still pursuing the path it opened . In providing the first evidence for electroweak theory, Gargamelle's results guided CERN to convert the Super Proton Synchrotron into a proton-antiproton collider powerful enough to enable the UA1 and UA2 collaborations to discover the W and Z bosons directly - a feat recognised by the award of the 1984 Nobel Prize in Physics to Carlo Rubbia and Simon van der Meer of CERN. During the 1990s, precision measurements of the W and Z bosons at the Large Electron-Positron collider confirmed important "quantum corrections" to electroweak theory (which, together with the theory of the strong force, quantum chromodynamics, makes up the Standard Model of particle physics). This guided physicists towards the discovery of the final piece of the electroweak jigsaw - the Higgs boson - at the Large Hadron Collider (LHC) in 2012, which led theorists François Englert and Peter Higgs to be awarded the 2013 Nobel Prize in Physics.
But the journey does not end there. As the LHC's ATLAS and CMS experiments continue to probe the Higgs boson and other mysterious sectors of the Standard Model at increasing levels of precision, physicists are investigating the feasibility of a successor collider at CERN - the proposed Future Circular Collider - that would go much further, opening the next chapter in electroweak exploration.