Prof. Leslie Leiserowitz first became intrigued by malaria when he was a young boy in South Africa. His father, who scouted the continent in search of wood for the family business, brought back not only tales of elephants and gorillas but also skin rashes and ringing in his ears, side effects of the quinine he took to prevent malaria. Decades later, while studying crystals at the Weizmann Institute of Science, Leiserowitz realized that malaria was in fact surprisingly pertinent to his research. He learned that the malaria parasite thrives inside red blood cells thanks to its knack for crafting crystals, and he set out to study these crystals, later joining forces with a chemistry faculty colleague, Prof. Michael Elbaum .
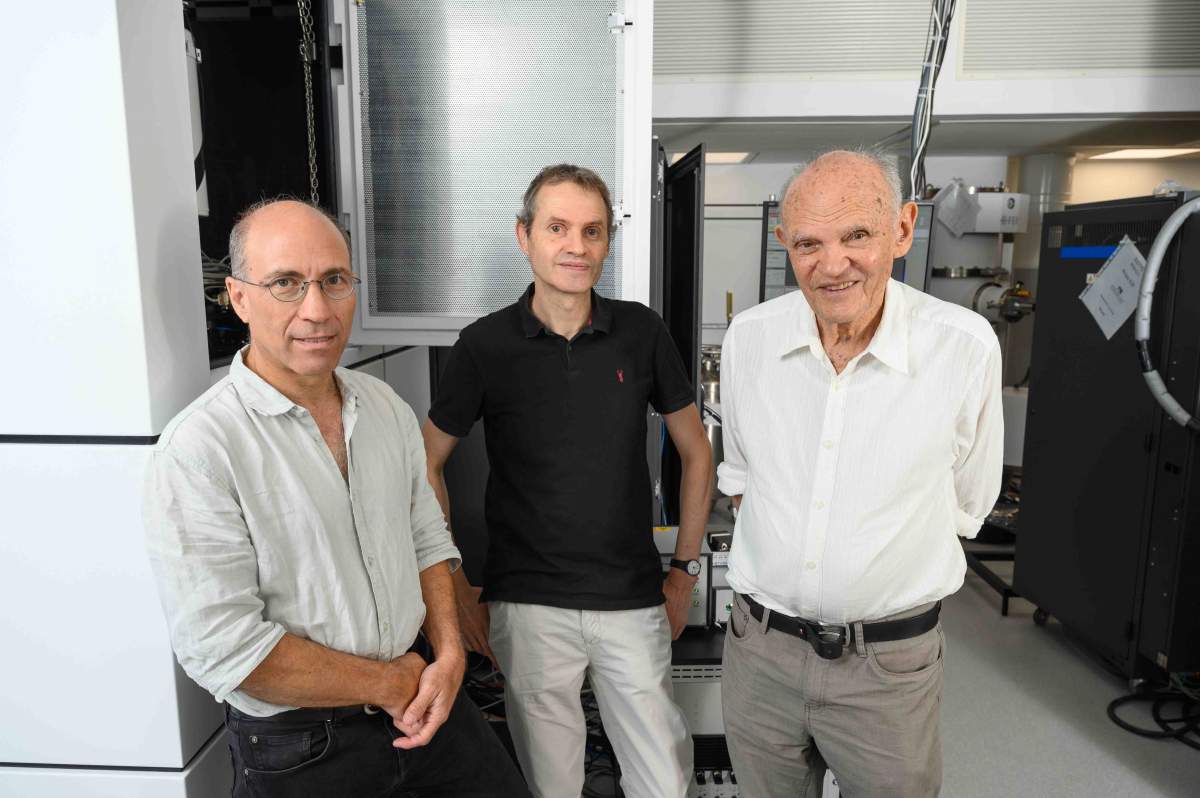
A new study - headed by Elbaum and Leiserowitz and conducted in collaboration with prominent research teams around the world - has culminated in a scientific paper that might help outwit the malaria parasite. It reveals in unprecedented detail the structure of crystals that the parasite builds in order to survive. Since most antimalarial drugs are thought to work by interfering with the formation and growth of these crystals, the new findings might lead to improved antimalarial medications.
"There had been enormous advances in imaging technologies such as electron and X-ray microscopy, and we realized that we could apply them to do something good for humanity," says Elbaum, explaining how this research came about. "It was an opportunity we simply couldn't pass up."
Seeing malaria pigment in a whole new way
Even though the incidence of malaria was drastically slashed in the first two decades of the 21st century, the disease remains an immense global health problem, killing more than half a million people each year, most of them young children. Much of the eradication effort is aimed at controlling the mosquitoes that, through their bites, transmit the malaria parasite - a single-celled organism belonging to the genus Plasmodium. Antimalarial drugs are also essential to this effort, but many of the existing medications have lost their effectiveness because the parasites have become resistant to them. Improved drugs could help break the cycle of the parasite's passage from mosquitoes to humans and back.
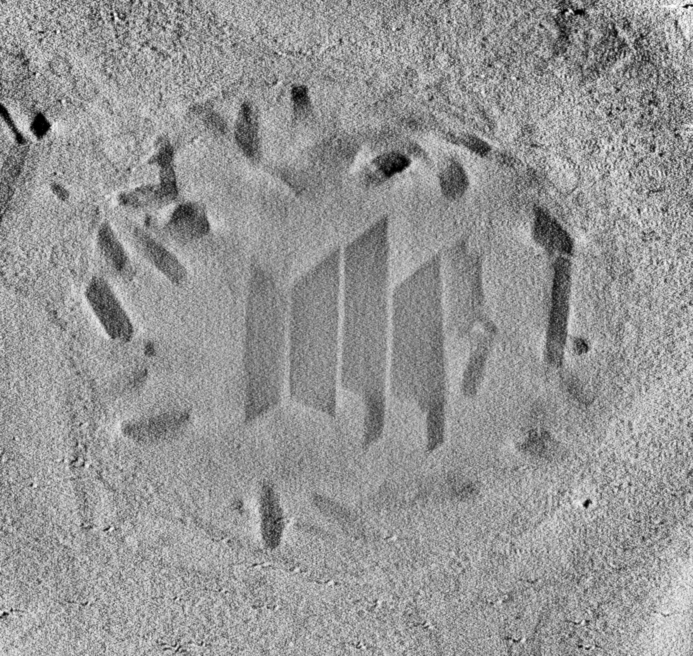
The production of crystals is a survival trick the parasite uses in its takeover of blood cells. This maneuver enables it to feast on hemoglobin, the oxygen-carrying protein in blood. Digesting the hemoglobin releases heme, an iron-containing molecular complex needed for binding oxygen. Freed from the surrounding protein, however, heme is so reactive that it can kill the parasite. That's precisely why Plasmodium pulls a survival stunt, in which it renders the heme harmless by packaging it into dark-colored crystals known as the malaria pigment, or more technically, as hemozoin. When it was discovered in the 19th century, hemozoin was initially thought to be made by the patient's body in response to the infection, but its true origin - through the doings of the parasite - was eventually understood.
In his early studies on hemozoin crystals, Leiserowitz was fascinated by their symmetries, a topic he had worked on for many years with his Weizmann colleague Prof. Meir Lahav. When applied to malaria, this topic becomes a life-and-death matter: The different ways in which heme molecules fit into the crystals not only create different symmetries but can also affect crystal growth, which, in turn, can seal the parasite's fate. However, such structural nuances were too subtle to be resolved by the methods of the day.
""There had been enormous advances in imaging technologies such as electron and X-ray microscopy, and we realized that we could apply them to do something good for humanity"
In the meantime, Elbaum had been independently working on Plasmodium from an entirely different angle. Together with colleagues at the Hebrew University of Jerusalem, he was studying Plasmodium cells as they go through their peculiar process of replication. Whereas most cells divide by splitting in two, the malaria parasite first makes numerous copies of its components within a red blood cell, then instantly divides into multiple daughter parasites that go on to infect new blood cells. When the scientists explored cellular nuclei during this process using newly available 3D electron microscopy methods, the hemozoin crystals also came into full view. So when Leiserowitz presented his work on these crystals at a faculty meeting, the collaboration with Elbaum was a natural outcome.

The collaboration proved fruitful from the start, thanks in large part to emerging new technologies for probing matter on the nanoscale. In their very first joint study, the scientists shed new light on crystal formation using soft X-ray tomography, a method that Elbaum had helped develop during a sabbatical in Berlin. Then, a new approach to cryo-electron tomography that Elbaum developed with Weizmann colleagues enabled the study of intact cells in which the malaria pigment is manufactured.
As luck would have it, a new lab working on the biology of the malaria parasite opened at Weizmann. It supplied Elbaum and Leiserowitz with infected red blood cells from which the hemozoin could be extracted. Revealing the structure of these natural crystals was crucial for medical applications, particularly because existing structural knowledge had been largely based on the more readily available synthetic crystals, used in most previous hemozoin studies.
But the crystals did not divulge their secrets easily. Searching for pieces of the structure puzzle that were still missing after a detailed three-dimensional analysis at Weizmann, Elbaum and Leiserowitz sent their pigment samples to colleagues at the University of Oxford and the Diamond Light Source (the UK's national synchrotron) who had installed a new method of electron crystallography that produced astounding images of the pigment. Having finished their initial analysis, the British scientists suggested securing the collaboration of researchers elsewhere.
"From then on, the research turned into a relay race of sorts, with each lab suggesting involving colleagues with top expertise in additional fields," Elbaum recalls. "The group finally expanded to a kind of all-star team for increasingly sophisticated analysis." Ultimately, the list of study authors came to comprise 17 researchers from Israel, the UK, Austria, the Czech Republic and the United States. That is, it took a coalition of some of the world's most advanced labs and a battery of the latest technologies to unravel the survival savvy honed over the course of evolution by a bunch of single-celled blood parasites.
Answering the question of the ugly crystals
The result of this international collaboration - a definitive, atom-by-atom three-dimensional structure of the malaria pigment - supplied a host of valuable insights. For starters, it solved a puzzle generated by previous studies, in which the Weizmann scientists had observed crystals of a peculiar trapezoid shape that resembled a kitchen cleaver: The "blade" end was always smooth and sharp, like a chisel, while the "handle" end was variable and often jagged.
"We were wondering how nature could produce something so ugly - these crystals looked as if they'd been bitten on one side," Leiserowitz recalls.
The detailed structure resolved the kitchen cleaver quandary. Heme molecules fit into the malaria pigment crystals in pairs, but because the "front" and "back" faces of these molecules differ chemically, they can pair up vis-à-vis one another in four distinct ways. In other words, there are four distinct heme building blocks, or basic units, of hemozoin crystals. Two of these are symmetric, but the other two are chiral, which means that they are mirror images of one another and cannot be superimposed, like the left and the right hand. When they grow together in a single crystal, the result can be an atomically disordered surface, including a jagged end. Such clear understanding of the crystal surfaces is essential to designing or evaluating drugs that must bind to the crystal to inhibit its growth.
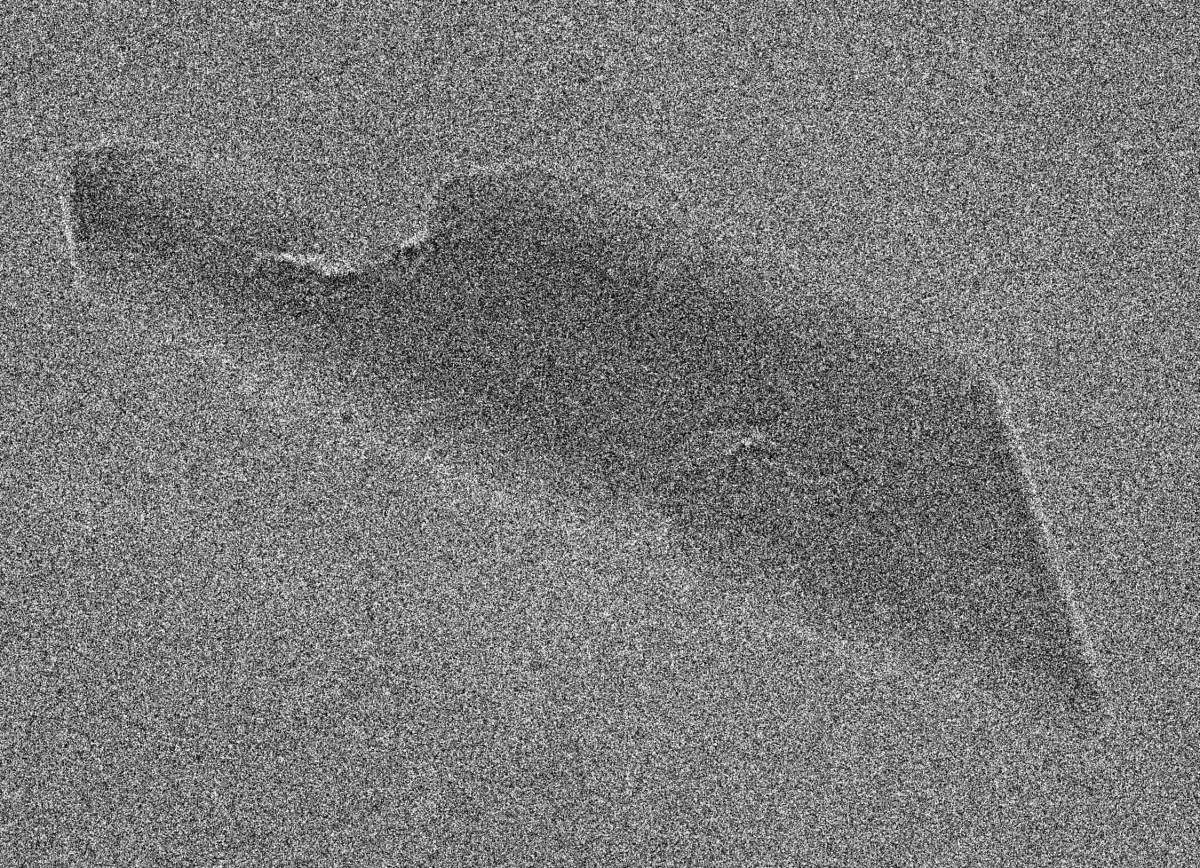
Drugs can attain their goal in ways that are more complex than stopping crystal growth, but the stoppage is vital for those other effects too. Leiserowitz explains the complexity using a car factory analogy: "Imagine that you are churning out cars, say, 500 a day, but at the end of the line, the drivers who have to take these cars away stop working, so all those cars pile up. That's exactly what happens when a drug prevents heme molecules from moving on to join a crystal. They pile up and jam the membranes, so nothing can get in or out, which helps to kill the parasite."
The study can facilitate the design of new drugs by making it much easier to, for example, calculate the interactions between crystals and the medication. In addition, the findings clarified which facets of the crystals grow more rapidly than others and identified facets whose growth is most likely to be inhibited by drug binding. Finally, the study revealed subtle but essential differences between natural and synthetic malaria crystals, which underscores the importance of designing future drugs on the basis of structural information about real-life crystals made by the parasite.
Elbaum recently presented the study's findings at the symposium "Leslie at 90: A Scientific Odyssey," held at Weizmann to mark Leiserowitz's 90th birthday. Of course, the publication of the paper coincided with this milestone birthday merely by chance, but it surely served as a grand reward for some two decades of Leiserowitz's research on the malaria pigment and for his life-long interest in malaria.
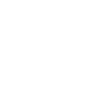
Science Numbers
The year 2022 saw nearly 250 million malaria cases and more than 600,000 malaria deaths occurring in 85 countries, according to estimates of the World Health Organization. Children under 5 are particularly vulnerable, accounting for about 4 out of 5 malaria deaths.
Each red blood cell contains about 1 billion heme molecules embedded in the hemoglobin. The smallest detectable crystals of malaria pigment, or hemozoin, contain about 20,000 heme molecules, but they can grow to contain tens of millions of heme molecules.The crystals range in length from about 100 to more than 500 nanometers. Since the wavelength of visible light starts at about 400 nanometers, scientists were already able to observe large malaria crystals or clusters of smaller ones with optical microscopes more than 100 years ago.
Elbaum is in Weizmann's Chemical and Biological Physics Department, and Leiserowitz, in the Molecular Chemistry and Materials Science Department. Study authors also included Dr. Paul Benjamin Klar of the University of Bremen, Germany; Dr. David Geoffrey Waterman of the Rutherford Appleton Laboratory, Oxfordshire, UK; Dr. Tim Gruene of the University of Vienna; Dr. Debakshi Mullick of Weizmann's Chemical and Biological Physics Department; Drs. Yun Song, James Boris Gilchrist and C. David Owen at the Diamond Light Source, Oxfordshire, UK; Prof. Peijun Zhang of the Diamond Light Source and the University of Oxford, UK; Dr. Wen Wen and Prof. Noa Marom of Carnegie Mellon University, Pittsburgh, PA, USA; Dr. Idan Biran of Weizmann's Molecular Chemistry and Materials Science Department; Dr. Lothar Houben of Weizmann's Chemical Research Support Department; Prof. Neta Regev-Rudzki of Weizmann's Biomolecular Sciences Department; Prof. Ron Dzikowski of the Hebrew University of Jerusalem; and Dr. Lukas Palatinus of the Institute of Physics of the Czech Academy of Sciences, Prague, Czech Republic.
In previous studies on the malaria pigment, Elbaum and Leiserowitz had collaborated with numerous other scientists who had made essential contributions. Among them were Dr. Sergey Kapishnikov, now at University College Dublin and SiriusXT, Dublin, Ireland, and Prof. Jens Als-Nielsen of the University of Copenhagen.
Elbaum developed cryogenic scanning transmission electron tomography (CSTET) together with Drs. Sharon G. Wolf and Lothar Houben of Weizmann's Chemical Research Support Department.
Prof. Michael Elbaum's research is supported by the Fritz Haber Center for Physical Chemistry, which he heads. Prof. Elbaum is the incumbent of the Sam and Ayala Zacks Professorial Chair.