A recent symposium on 'The complex life of RNA' brought together scientists from across the world interested in exploring one of the most crucial molecules essential to life
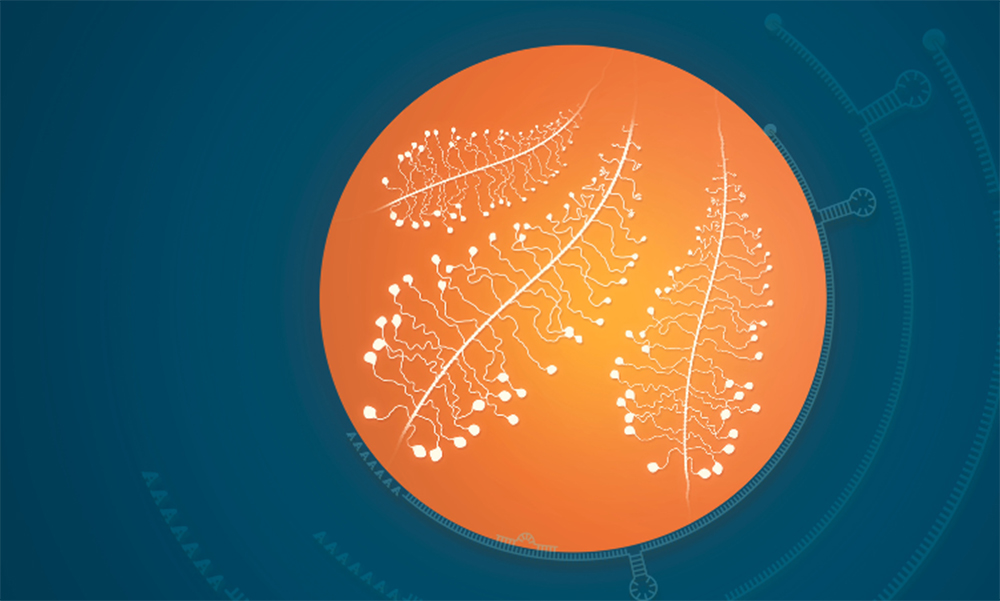
By Sepideh Mohammadi Koubjari
Scientists from around the world gathered at EMBL Heidelberg from 15-18 October 2024 to share their latest discoveries about RNA - a molecule that's proven crucial for life and modern medicine. Here are five points emerging from the conference that I'd like us to explore together.
1. The tale of the RNA tail
RNAs have several ways to protect themselves. One is a long stretch of chemical groups called 'adenines' at their very end. Lori Passmore from the MRC Laboratory of Molecular Biology, UK, talked about how, why, and where cells control their RNA tails. She showed that specialised proteins can act like molecular tethers, promoting the shortening of these tails - a process called deadenylation. This process can be adjusted through a chemical modification called phosphorylation, which acts like a molecular dimmer switch.
On a related note, Bahareh Torkzaban from Johns Hopkins University, USA, introduced a promising therapeutic approach called 'mRNA Booster' that can potentially increase the production of specific proteins in cells. It involves attaching a long adenine tail to messenger RNA molecules, which could help treat diseases where patients don't produce enough essential proteins, allowing doctors to adjust treatment levels as needed.
2. The protein partners of RNA
RNA rarely works alone in cells - it partners with proteins and other RNAs to get business done. Andreas Mayer from the Max Planck Institute for Molecular Genetics, Germany, provided interesting insights into how RNA and its protein partners behave during cell differentiation - the process by which cells become specialised to perform certain functions.
His team studied how RNA molecules change during development. RNA can exist in different versions (isoforms), and each form partners with specific proteins. These partnerships are dynamic - they change based on which proteins are available at a particular developmental stage. The RNA isoforms and their protein interactions can change what types of proteins are made in the cell and how they function.
Juan Valcárcel Juárez from the Centre for Genomic Regulation (CRG), Spain, studied how cells process RNA using a complex molecular machine called the spliceosome, which acts like an editor to cut and join together pieces of genetic information. Their research revealed that different parts of this cellular machinery have specialised roles in deciding which pieces to keep or remove - like having different editors (copyeditor, subeditor, etc.) responsible for specific aspects of editing. This discovery helps us better understand how cells control their genetic messages in health and disease.
Hervé Le Hir from École normale supérieure, France, studied protein complexes called EJCs (Exon Junction Complexes) that attach to mRNA molecules after they're processed. Using a new glowing tag system, they shed light on how about one-third of these EJCs need protein production (translation) to be removed from the RNA - suggesting that the other two-thirds are removed through different mechanisms. They also discovered how these complexes play a crucial role in directing RNA messages to cellular structures called centrosomes and basal bodies, revealing a new role for EJCs in directing RNA traffic within cells.
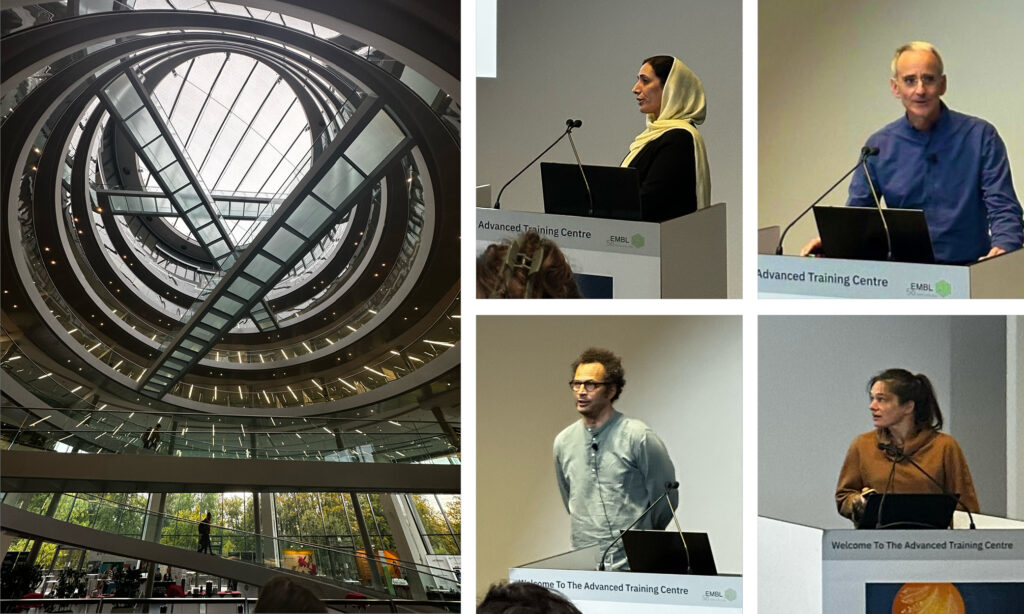
3. Artificial intelligence meets RNA research
Just as AI is transforming many aspects of our lives, it's also changing how scientists study RNA. Researchers are now using AI tools similar to those behind ChatGPT to predict how RNA molecules fold and function in cells. For instance, Yiliang Ding's team at John Innes Centre, UK, has developed PlantRNA-FM, an AI tool that helps scientists understand RNA structure in plants.
The famous Nobel Prize-winning AlphaFold system, which made headlines for predicting the structure of nearly every protein known to science, is also now being adapted to understand how RNA interacts with proteins. Julius Brennecke's team at the Institute of Molecular Biotechnology, Austria, used AlphaFold 3, the latest version of the AI system, to study how proteins interact with small RNA molecules called piRNAs in gene silencing. This application of AI is helping scientists understand complex molecular interactions that would be difficult to study through traditional methods alone.
4. Scientists question what they know
Sometimes, the most valuable scientific insights come from questioning what we take for granted. Maria Hondele from the University of Basel, Switzerland, warned scientists about a widespread laboratory practice - adding fluorescent tags to molecules to track them under the microscope. Her team discovered that these tags could change how molecules behave in cells. This highlights the importance of critical thinking in scientific research and the need to constantly reevaluate and refine our methods.
Hervé Seitz from CNRS, France, challenged how microRNAs (small RNA molecules that help control gene activity, another Nobel Prize-winning discovery ) can interact with thousands of different target genes in our cells. His research shows that many of these predicted interactions might not be as important as previously thought. When certain microRNAs naturally disappeared during evolution, most of their supposed target genes stayed unchanged. This discovery suggests that far fewer microRNA interactions are truly essential for biological functions than scientists initially believed.
5. RNA as a drug target
Matthew Disney from The Wertheim UF Scripps Institute, USA, presented pioneering research on targeting RNA with small molecules. His team studies how RNA structures fold and function, aiming to develop new medicines that can directly interact with disease-causing RNA molecules. Most drugs target proteins, but Disney's approach focuses on identifying specific patterns in RNA structures that small molecules can recognise and bind to, potentially treating diseases that have been difficult to address with traditional medicine. They can now identify promising RNA targets across the human genome and design molecules that bind to these targets and trigger the degradation of disease-causing RNAs.
What Does This Mean for You?
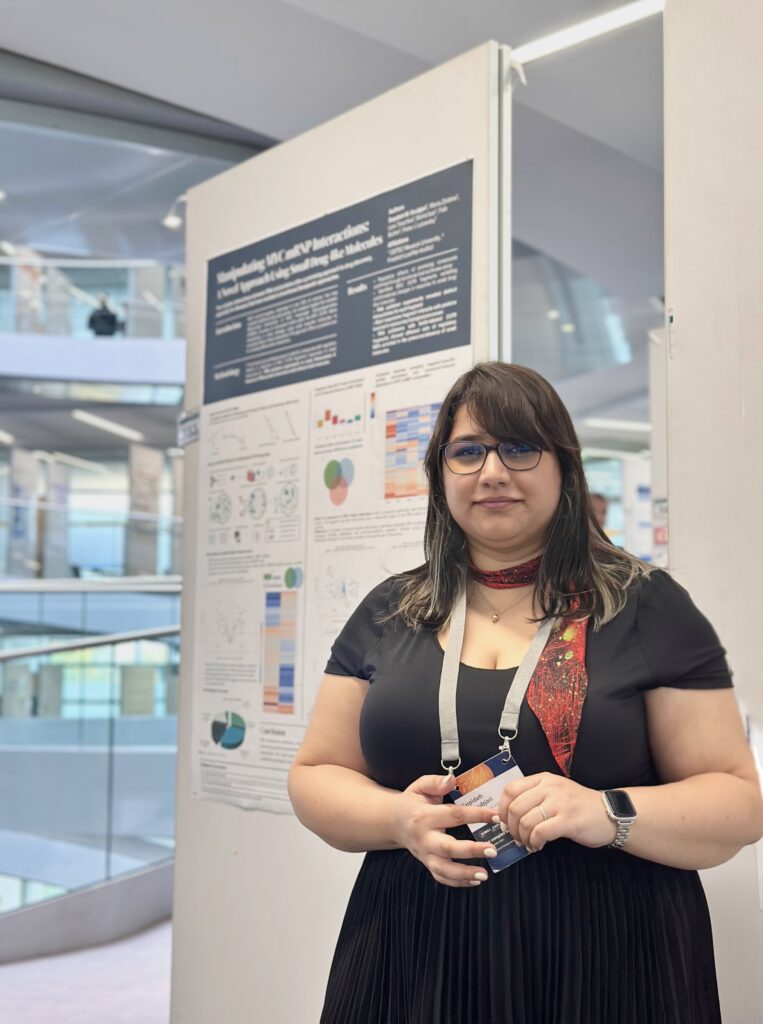
While these findings might seem abstract, they have real-world implications. Just as RNA research led to effective COVID-19 vaccines , continuing research in this area renders goals like targeted therapies against cancer and rare genetic diseases more achievable. This global research effort and open sharing of discoveries, such as what I witnessed at the EMBL conference, is transforming how we treat diseases as well as advancing our understanding of life's fundamental processes. Ultimately, understanding how RNA works helps us grasp the complexities of cellular life.
The author, Sepideh Mohammadi Koubjari, is a PhD student at the Central European Institute of Technology, Masaryk University and part of the SciCanvas startup team. She attended the EMBO | EMBL Symposium 'The complex life of RNA' as an event reporter as well as an RNA researcher.