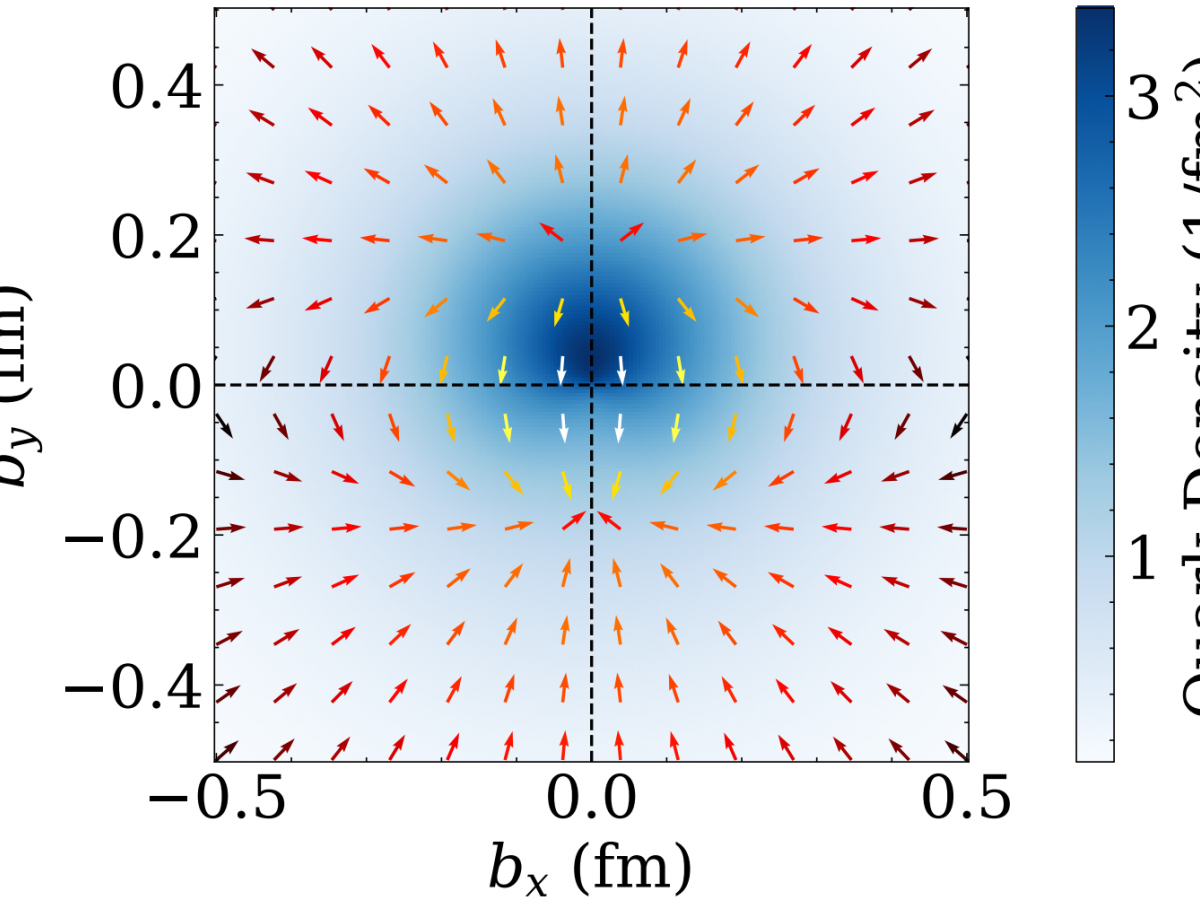
New force distributions revealed by the calculations, on top of the probability map of finding a quark in the proton. Joshua Crawford / University of Adelaide.
Scientists have now mapped the forces acting inside a proton, showing in unprecedented detail how quarks-the tiny particles within-respond when hit by high-energy photons.
The international team includes experts from the University of Adelaide who are exploring the structure of sub-atomic matter to try and provide further insight into the forces that underpin the natural world.
"We have used a powerful computational technique called lattice quantum chromodynamics to map the forces acting inside a proton," said Associate Professor Ross Young, Associate Head of Learning and Teaching, School of Physics, Chemistry and Earth Sciences, who is part of the team.
"This approach breaks down space and time into a fine grid, allowing us to simulate how the strong force-the fundamental interaction that binds quarks into protons and neutrons-varies across different regions inside the proton."
The team's result is possibly the smallest-ever force field map of nature ever generated. They have published their findings in the journal Physical Review Letters.
University of Adelaide PhD student, Joshua Crawford's calculations led the work together with the University of Adelaide team and international collaborators.
"Our findings reveal that even at these minuscule scales, the forces involved are immense, reaching up to half a million Newtons, the equivalent of about 10 elephants, compressed within a space far smaller than an atomic nucleus," said Joshua.
"These force maps provide a new way to understand the intricate internal dynamics of the proton, helping to explain why it behaves as it does in high-energy collisions, such as those at the Large Hadron Collider, and in experiments probing the fundamental structure of matter."
The Large Hadron Collider (LHC) is the world's largest and highest-energy particle accelerator. It was built by the European Organization for Nuclear Research (CERN) in collaboration with over 10,000 scientists and hundreds of universities and laboratories across more than 100 countries. The LHC's goal is to allow physicists to test the predictions of different theories of particle physics.
"Edison didn't invent the light bulb by researching brighter candles-he built on generations of scientists who studied how light interacts with matter," said Associate Professor Young.
"In much the same way, modern research such as our recent work is revealing how the fundamental building blocks of matter behave when struck by light, deepening our understanding of nature at its most basic level.
"As researchers continue to unravel the proton's inner structure, greater insight may help refine how we use protons in cutting-edge technologies.
"One prominent example is proton therapy, which uses high-energy protons to precisely target tumours while minimising damage to surrounding tissue.
"Just as early breakthroughs in understanding light paved the way for modern lasers and imaging, advancing our knowledge of proton structure could shape the next generation of applications in science and medicine.
"By making the invisible forces inside the proton visible for the first time, this study bridges the gap between theory and experiment-just as earlier generations uncovered the secrets of light to transform the modern world."