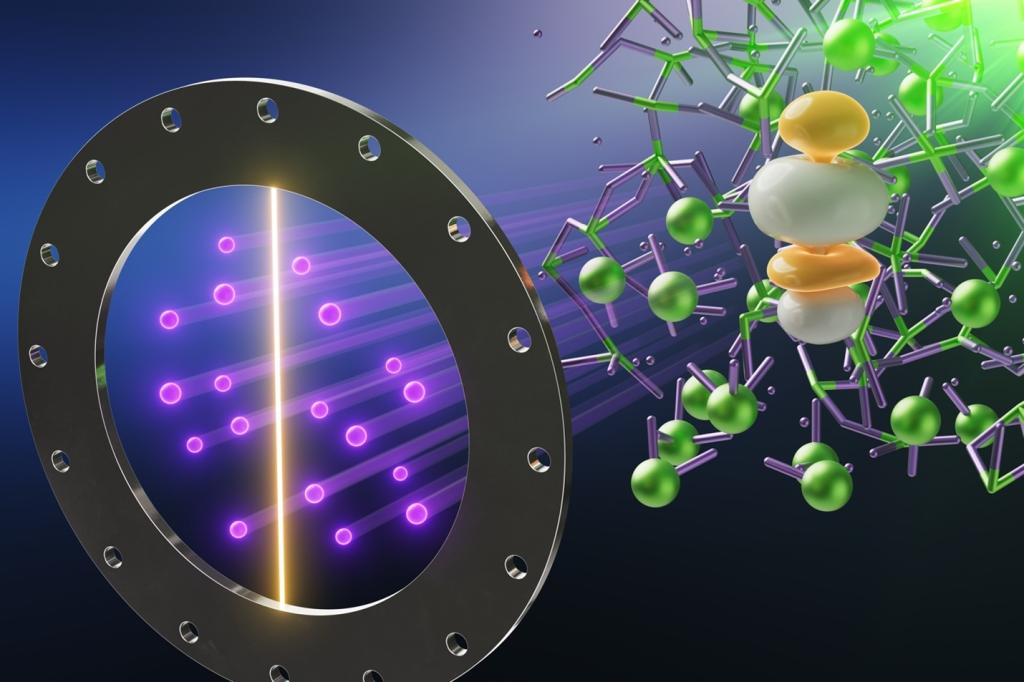
The Department of Energy's Oak Ridge National Laboratory is a world leader in molten salt reactor technology development - and its researchers additionally perform the fundamental science necessary to enable a future where nuclear energy becomes more efficient. In a recent paper published in the Journal of the American Chemical Society, researchers have documented for the first time the unique chemistry dynamics and structure of high-temperature liquid uranium trichloride (UCl3) salt, a potential nuclear fuel source for next-generation reactors.
"This is a first critical step in enabling good predictive models for the design of future reactors," said ORNL's Santanu Roy, who co-led the study. "A better ability to predict and calculate the microscopic behaviors is critical to design, and reliable data help develop better models."
For decades, molten salt reactors have been expected to possess the capacity to produce safe and affordable nuclear energy, with ORNL prototyping experiments in the 1960s successfully demonstrating the technology. Recently, as decarbonization has become an increasing priority around the world, many countries have re-energized efforts to make such nuclear reactors available for broad use.
Ideal system design for these future reactors relies on an understanding of the behavior of the liquid fuel salts that distinguish them from typical nuclear reactors that use solid uranium dioxide pellets. The chemical, structural and dynamical behavior of these fuel salts at the atomic level are challenging to understand, especially when they involve radioactive elements such as the actinide series - to which uranium belongs - because these salts only melt at extremely high temperatures and exhibit complex, exotic ion-ion coordination chemistry.
The research, a collaboration among ORNL, Argonne National Laboratory and the University of South Carolina, used a combination of computational approaches and an ORNL-based DOE Office of Science user facility, the Spallation Neutron Source, or SNS, to study the chemical bonding and atomic dynamics of UCl3 in the molten state.
The SNS is one of the brightest neutron sources in the world, and it allows scientists to perform state-of-the-art neutron scattering studies, which reveal details about the positions, motions and magnetic properties of materials. When a beam of neutrons is aimed at a sample, many neutrons will pass through the material, but some interact directly with atomic nuclei and "bounce" away at an angle, like colliding balls in a game of pool.
Using special detectors, scientists count scattered neutrons, measure their energies and the angles at which they scatter, and map their final positions. This makes it possible for scientists to glean details about the nature of materials ranging from liquid crystals to superconducting ceramics, from proteins to plastics, and from metals to metallic glass magnets.
Each year, hundreds of scientists use ORNL's SNS for research that ultimately improves the quality of products from cell phones to pharmaceuticals - but not all of them need to study a radioactive salt at 900 degrees Celsius, which is as hot as volcanic lava. After rigorous safety precautions and special containment developed in coordination with SNS beamline scientists, the team was able to do something no one has done before: measure the chemical bond lengths of molten UCl3 and witness its surprising behavior as it reached the molten state.
"I've been studying actinides and uranium since I joined ORNL as a postdoc," said Alex Ivanov, who also co-led the study, "but I never expected that we could go to the molten state and find fascinating chemistry."
What they found was that, on average, the distance of the bonds holding the uranium and chlorine together actually shrunk as the substance became liquid - contrary to the typical expectation that heat expands and cold contracts, which is often true in chemistry and life. More interestingly, among the various bonded atom pairs, the bonds were of inconsistent size, and they stretched in an oscillating pattern, sometimes achieving bond lengths much larger than in solid UCl3 but also tightening to extremely short bond lengths. Different dynamics, occurring at ultra-fast speed, were evident within the liquid.
"This is an uncharted part of chemistry and reveals the fundamental atomic structure of actinides under extreme conditions," said Ivanov.
The bonding data were also surprisingly complex. When the UCl3 reached its tightest and shortest bond length, it briefly caused the bond to appear more covalent, instead of its typical ionic nature, again oscillating in and out of this state at extremely fast speeds - less than one trillionth of a second.
This observed period of an apparent covalent bonding, while brief and cyclical, helps explain some inconsistencies in historical studies describing the behavior of molten UCl3. These findings, along with the broader results of the study, may help improve both experimental and computational approaches to the design of future reactors.
Moreover, these results improve fundamental understanding of actinide salts, which may be useful in tackling challenges with nuclear waste, pyroprocessing. and other current or future applications involving this series of elements.
The research was part of DOE's Molten Salts in Extreme Environments Energy Frontier Research Center, or MSEE EFRC, led by Brookhaven National Laboratory. The research was primarily conducted at the SNS and also used two other DOE Office of Science user facilities: Lawrence Berkeley National Laboratory's National Energy Research Scientific Computing Center and Argonne National Laboratory's Advanced Photon Source. The research also leveraged resources from ORNL's Compute and Data Environment for Science, or CADES.
UT-Battelle manages ORNL for DOE's Office of Science, the single largest supporter of basic research in the physical sciences in the United States. The Office of Science is working to address some of the most pressing challenges of our time. For more information, please visit energy.gov/science. - Emily Tomlin