When we plug in our cell phones to charge them, we take it for granted that they'll soon be brimming with energy for scrolling, texting and receiving news alerts. But the technology powering this - rechargeable lithium-ion batteries - heralded a genuine technological revolution when these batteries first appeared on the commercial scene in the 1990s, and they earned their developers the Nobel Prize in Chemistry in 2019. Without this innovation, our smartphones, wireless headphones and electric vehicles would be both environmentally and economically infeasible.
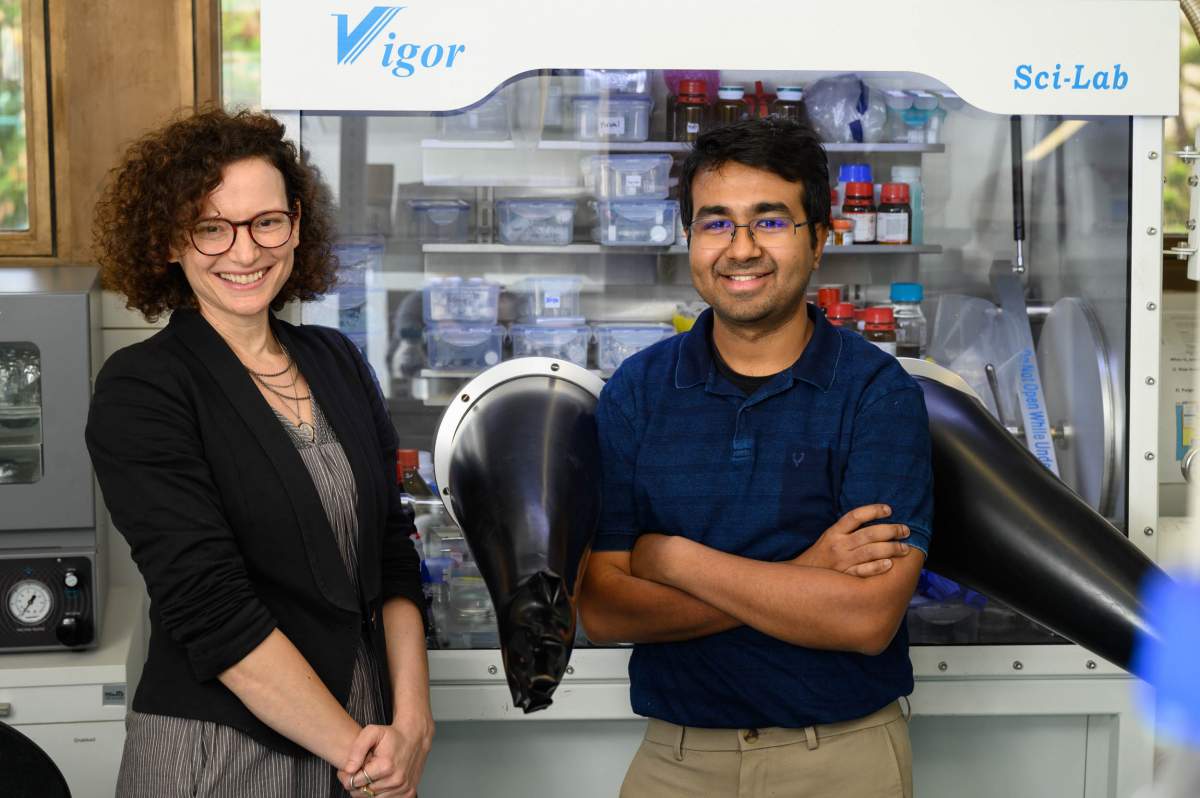
The rate at which technology is advancing calls for stronger and safer batteries, but developing them is no easy task. Lithium metal batteries, for example, could in the future provide significantly more energy than those in common use today, but they also pose a significant challenge: During every charge, tiny threads called dendrites are formed within them. When dendrites accumulate, they can create metallic bridges inside the battery, enabling an uncontrolled transfer of electrons that is liable to ruin the battery and, more worryingly, create a fire hazard. Until now, researchers had limited techniques available to characterize the formation of dendrites. In a new study undertaken in the laboratory of Prof. Michal Leskes , from the Weizmann Institute of Science's Molecular Chemistry and Materials Science Department, researchers led by Dr. Ayan Maity developed an innovative technique that allows them to not only identify what within the battery affects the accumulation of dendrites but also quickly inspect the effectiveness and safety of alternative battery components.
Rechargeable batteries work by enabling positively charged ions to move from the negative electrode (the anode) to the positive one (the cathode) through an electrically conductive substance called the electrolyte. When the battery is being charged, the ions return to the anode - contrary to what naturally happens in a chemical reaction - and this prepares the battery for repeated use. Lithium metal batteries are innovative in that their anodes are made of pure lithium metal, which allows them to store large quantities of energy. The problem is that lithium metal is highly active chemically and interacts with any material it encounters. So, when it interacts with the electrolyte, dendrites are quickly created in quantities that endanger the user and the health of the battery.
The fire hazard can be avoided by replacing the liquid, flammable electrolyte in the battery with a solid, nonflammable material, such as a composite of polymers and ceramic particles. The balance between these two components significantly affects the formation of dendrites, but the main challenge remains finding the ideal composition to extend the lifespan of the batteries.
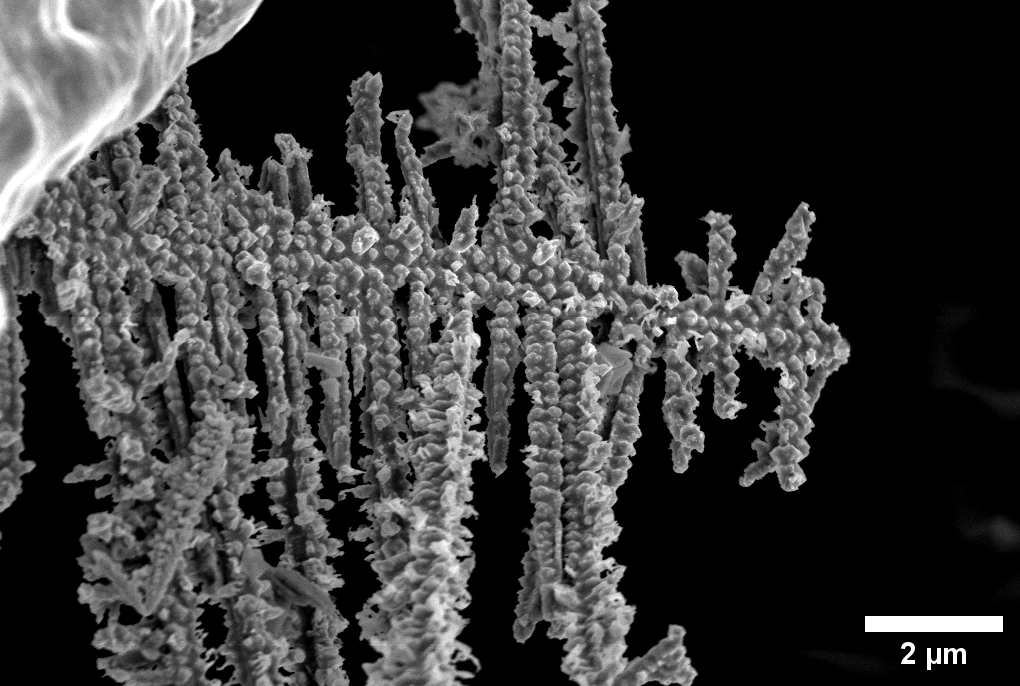
The research team decided to address this question by using nuclear magnetic resonance (NMR) spectroscopy - an accepted technique for revealing the chemical structure of material - which allowed them to follow the development of dendrites and identify chemical interactions within the electrolyte. "When we examined the dendrites in batteries with differing ratios of polymer and ceramic, we found a kind of 'golden ratio': Electrolytes that are composed of 40 percent ceramic had the longest lives," Leskes explains. "When we went above 40 percent ceramic, we encountered structural and functional problems that impeded battery performance, while less than 40 percent led to reduced battery life." Surprisingly, however, in the batteries that performed best, the number of dendrites was increased, but their growth was blocked and they formed fewer of these dangerous bridges.
""We started with a purely scientific question that had nothing to do with dendrites, and this led us to a study with practical applications that could improve everybody's life"
These findings led the researchers to the million-dollar question, which could be worth a lot more than that in terms of commercial applications: What is blocking the growth of the dendrites? The researchers hypothesized that the answer lay in a thin layer on the surface of the dendrites known as the solid electrolyte interphase or SEI. The SEI layer, which forms when the dendrites react with the electrolyte, can be composed of various substances that have either a positive or negative effect on the battery. For example, the chemical composition of the SEI layer can hinder or improve the movement of lithium ions along the battery and block or facilitate the movement of harmful materials from the anode to the cathode, which in turn can impede or accelerate the development of dendrites.
To characterize SEI layers, the researchers needed to think "outside the battery." Because these layers are composed of a just a few dozen nanometers of atoms, the signals picked up from them by the NMR are fairly weak. In an effort to bolster the signals, the researchers resorted to a technique that is rarely used in the study of batteries: enhancing the NMR by means of dynamic nuclear polarization.
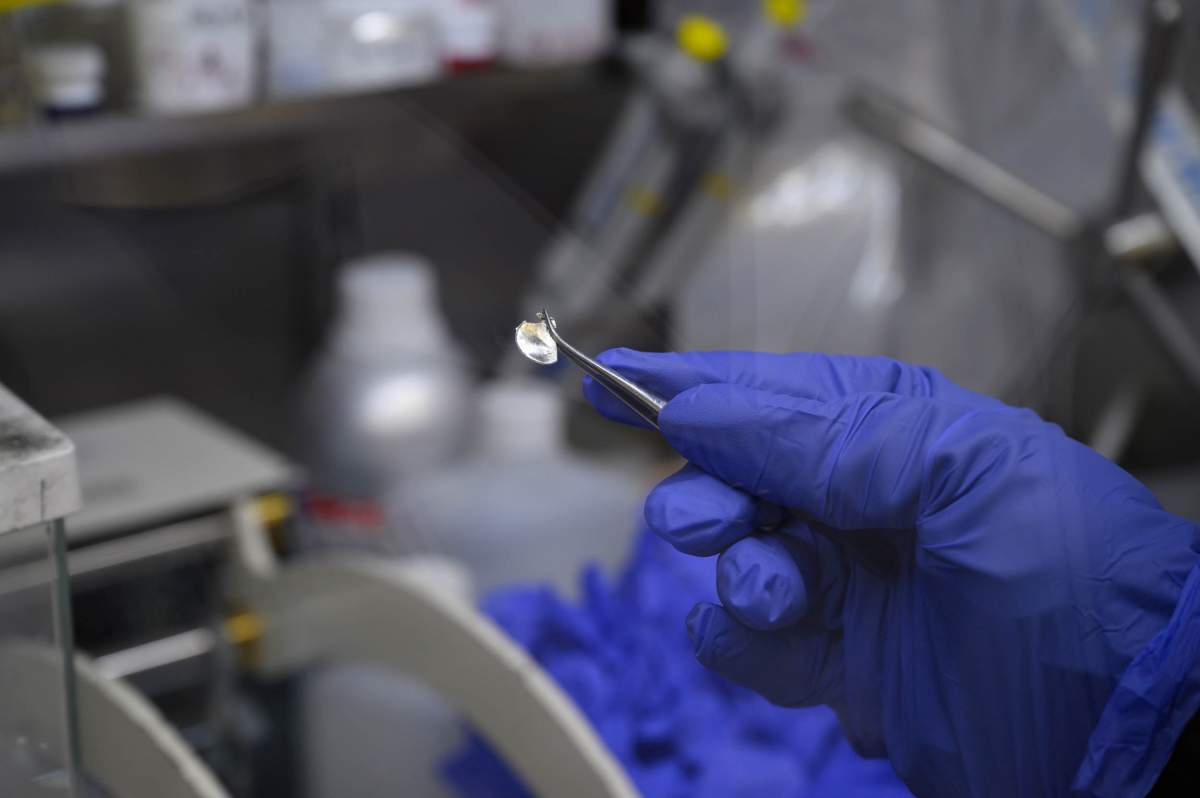
This technique makes use of the strong spin of polarized lithium electrons, which send out powerful signals that intensify the signals emitted by the atomic nuclei in the SEI layer. By using this technique, the researchers were able to reveal the precise chemical composition of the SEI layer, which helped them uncover the interactions that occur between the lithium and the various structures in the electrolyte. They were able, for example, to work out whether a dendrite had developed during the interaction of the lithium with the polymer or with the ceramic. This also led to the surprising discovery that SEI layers created on dendrites sometimes make the transfer of ions within the electrolyte more efficient while also blocking dangerous substances.
The findings of the study provide new insights that could be used to develop sturdier, stronger and safer batteries capable of supplying more energy at a lower environmental and economic cost. These future batteries will be able to power larger and smarter devices without having to increase the size of the battery, while simultaneously extending its lifespan.
"One of the things I love most about this study is that, without a profound scientific understanding of fundamental physics, we would not have been able to understand what happens inside a battery. Our process was very typical of the work here at the Weizmann Institute. We started with a purely scientific question that had nothing to do with dendrites, and this led us to a study with practical applications that could improve everybody's life," Leskes says.
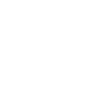
Science Numbers
The SEI layer in a battery is no more than 5 to 50 nanometers thick - around 1/1000 of the thickness of a human hair - but it still contains complex chemical structures. Using NMR spectroscopy, it would have taken years to understand the chemical composition of the SEI layer, but, using dynamic nuclear polarization, the task was completed in just hours.
Also participating in the study were Dr. Asya Svirinovsky-Arbeli, Yehuda Buganim and Chen Oppenheim from Weizmann's Molecular Chemistry and Materials Science Department.
Prof. Michal Leskes heads the Solo Dwek and Maurizio Dwek Research School of Chemical Science and the Tom and Mary Beck Center for Renewable Energy, which is part of the Institute for Environmental Sustainability (IES). Her research is supported by the Adolfo Eric Labi Fund for Research on High-Energy Storage Systems; the Henry Chanoch Krenter Institute for Biomedical Imaging and Genomics; the Henri Gutwirth Award; and the Sagol Weizmann-MIT Bridge Program.