The phase and the group velocity of light propagating in conventional optical media cannot exceed the speed of light in vacuum.
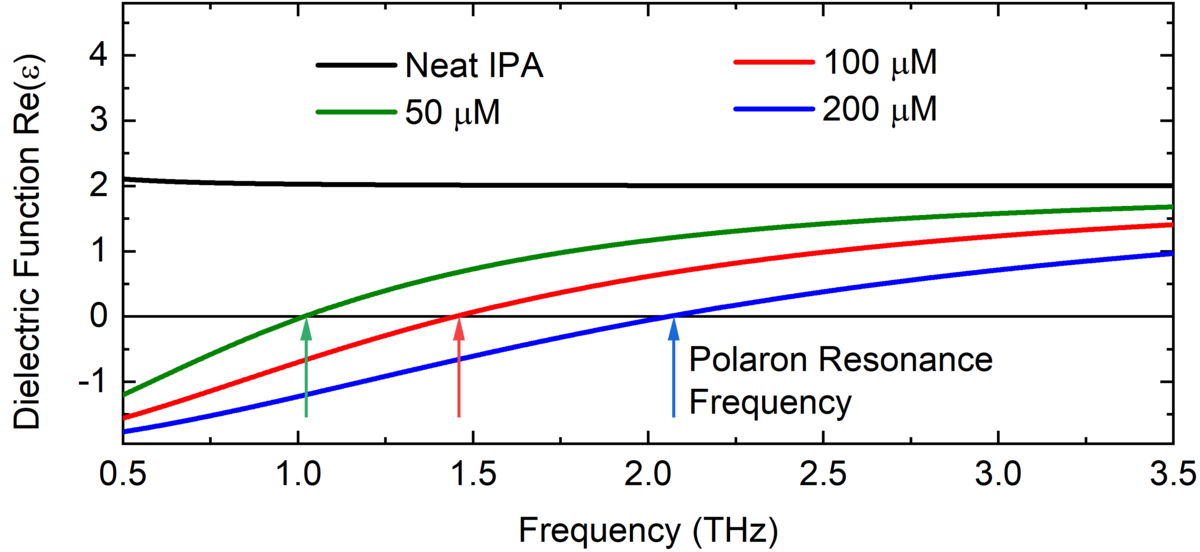
Fig. 1. Real part of the dielectric function of isopropanol (IPA) without optical excitation (black line) and with optical excitation leading to the three different electron concentrations of 50, 100 and 200 µM (colored lines). The frequency at which the curves intersect with the zero line gives the polaron resonance frequency. | Fig.: Runge, MBI
However, in so-called epsilon-near-zero (ENZ) materials, light exhibits an infinite phase velocity and a vanishing group velocity for a particular color (frequency). So far, such properties were only observed in very few solids and nano-engineered materials. The present work opens a completely new avenue by transiently turning ordinary liquids, such as water and alcohols, into ENZ materials at terahertz (THz, 1 THz = 1012 Hz) frequencies through the interaction with intense femtosecond laser pulses.
Ionization of a polar molecular liquid with femtosecond laser pulses generates free electrons, which localize or 'solvate' on a femtosecond time scale and eventually occupy voids in the network of molecules, a disordered array of electric dipoles. The binding energy of the electron in its final location is mainly determined by electric forces between the electron and the molecular dipoles of the liquid. During the ultrafast localization process, the electric coupling allows for kicking off collective oscillations of the electron and thousands of liquid molecules close-by. This many-body excitation is called polaron and displays a distinct frequency in the THz range, determined by the concentration of electrons in the liquid. At the polaron frequency, the dielectric function and/or the refractive index of the liquid crosses the zero line, as shown in Fig. 1. In other words, the phase velocity of light at this frequency approaches infinity and the group velocity of light pulses should go to zero, a behavior characteristic for an ENZ material.
Researchers from the Max Born Institute in Berlin and Tulane University in New Orleans have now demonstrated that polar liquids containing solvated electrons represent a new class of ENZ materials with tunable light propagation properties. In the current issue of Physical Review Letters ( doi.org/10.1103/PhysRevLett.134.056901 ), they report results from experiments, in which electrons in a polar liquid have first been generated by femtosecond optical ionization and the propagation of short THz pulses in this medium with a polaron frequency of some 1.5 THz has been followed in a time-resolved way. The experimental method gives insight in the THz electric field, thus revealing both phase and group velocities of the propagating THz pulses (Fig. 2). Both phase and group velocities are strongly modified compared to the neat liquid and the pulse envelope is reshaped, that means broadened. This behavior becomes most obvious when comparing the propagation of the transmitted THz pulse (red lines) below and above the polaron resonance to the THz pulses propagated through vacuum (blue lines) and the unexcited ordinary liquid (black lines). Such properties are a hallmark of ENZ behavior and in line with theoretical calculations.
For applications, a shift of the polaron frequency by a simple change of electron concentration represent a most appealing feature, which allows a controlled tailoring of the material's ENZ properties in a frequency range from approximately 0.1 to 10 THz. These findings pave the way for new techniques of controlling light propagation in liquids, possibly allowing for advances is optical sensing and communication.
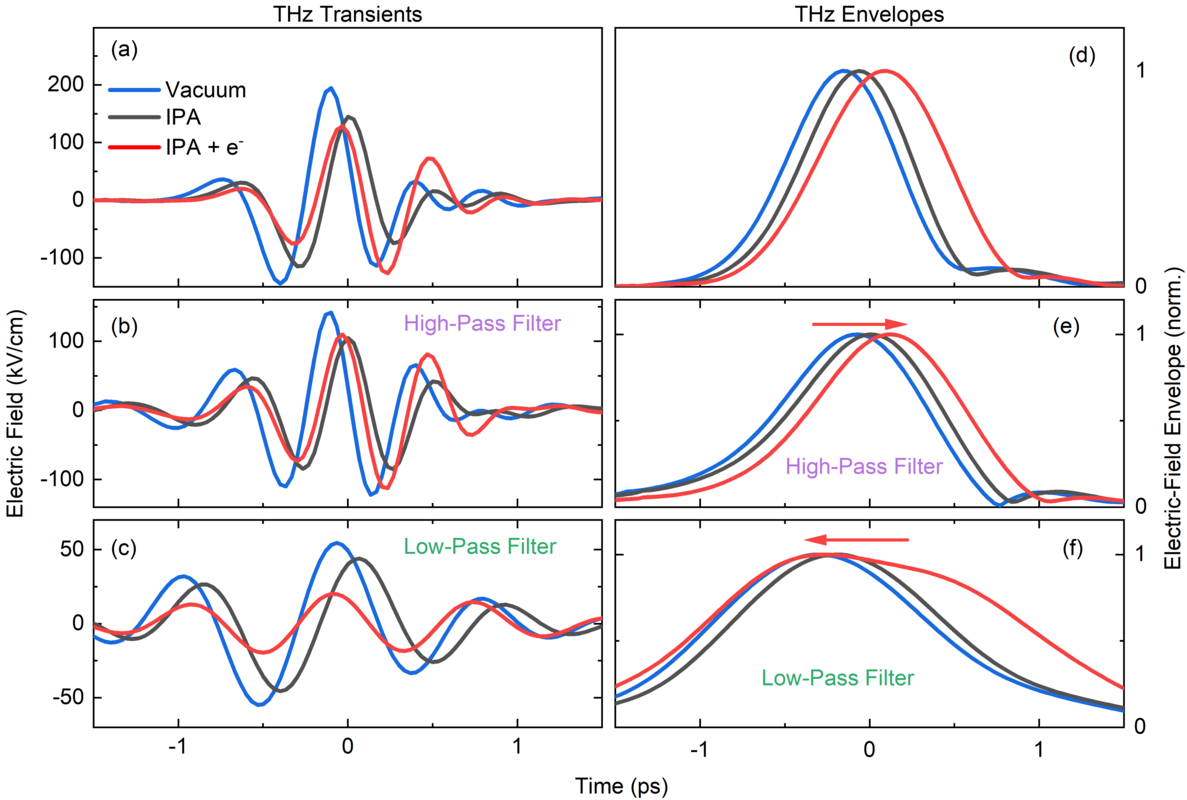
M. Runge, M. Woerner, D. I. Bondar, T. Elsaesser
Phys. Rev. Lett. 134, 056901 (2025)