A team of physicists has developed a powerful new way of simulating strange magnetic structures known as skyrmions--microscopic whirlpools of spinning electrons - that could transform data storage.
A study describing the modelling effort was published in the journal Physical Review X on March 11.
Every electron behaves like a tiny bar magnet, and the direction it "points" in is called its spin direction. In many magnetic materials, the spins of electrons all point in the same direction - like compass needles lining up. Physicists describe this phenomenon as "collinear spin texture."
But in some materials, the spins point in different directions at different places, forming patterns like spirals, vortices, or swirls. These are called noncollinear spin textures. These arrangements often emerge in materials with special symmetries or interactions - and they can lead to unusual and useful behaviors.
"Imagine a landscape where each blade of grass bends in a different direction, forming spirals and swirls," said lead author Hsiao-Yi Chen, with the Institute of Material Research at Tohoku University. "That is sort of what happens with electron spins inside certain magnetic materials."
Complicating things even further, if all spins lie in one plane, physicists call this coplanar noncollinear, while if spins point in three dimensions, then this is noncoplanar noncollinear.
Noncoplanar noncollinear spin textures are especially interesting because they can give rise to something called the topological Hall effect.
The Hall effect is a classic physics phenomenon: when you run electricity through a material in the presence of a magnetic field, the moving electrons get nudged sideways, creating a voltage across the material. The topological Hall effect is, well, a twist on that. Instead of needing an external magnet, the sideways motion happens because of the internal twists and turns of the electron spins themselves.
And this effect is especially strong in materials that host skyrmion lattices--tiny, stable magnetic whirlpools that arrange themselves in regular patterns. These skyrmions can be just a few nanometers wide. But skyrmions are also robust enough that they're being explored as the building blocks of future low-energy, high-density memory technologies.
Traditionally, memory storage in electronic devices encodes information in the charge of electrons. But in the emerging field of "spintronics," the spin might be able to perform such storage instead. One of the bottlenecks in realizing spintronic devices has been identification of suitable materials. One group of promising candidates are noncoplanar spin magnets, but our understanding of their electronic structure and material properties has remained limited.
In order to improve such understanding, engineers first need to be able to simulate how electrons move through the complex, twisted environment of skyrmion lattices, and this has proved to be a major computational challenge. The usual method for such simulations, a technique called density functional theory (DFT), is a powerful way to predict the properties of complex spin textures. But DFT is also computationally prohibitive due to the large system sizes involved, making such calculations in most cases impractical.
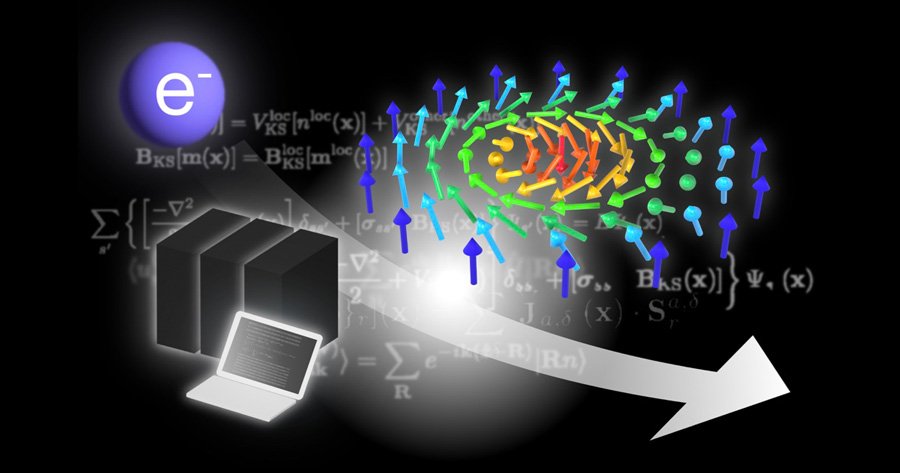
However, the research team's new approach sidesteps these issues in quantum simulations. Rather than modeling every single twist and turn across an enormous lattice, the researchers developed a shortcut: they first trained a model to recognize how local spin swirls affect electrons, and then used what they call a "tight-binding" framework to zoom out and simulate entire materials efficiently.
Using this method, they successfully modeled the behavior of electrons in a noncoplanar spin magnet material called Gd₂PdSi₃, which is known to host skyrmion lattices, and found that their computational predictions closely matched experimental measurements.
The team's results not only provide new insights into the fundamental physics of skyrmions but also offer a powerful tool for identifying and designing new materials where these topological effects could be harnessed.
And the team is already moving forward with this hunt for optimal spintronic materials. Controlling spin is another key issue in spintronic technology, and it is currently achieved using spin waves--a collective spin rotation, another quantum effect that is poorly understood.
- Publication Details:
Title: Topological Hall effect of Skyrmions from first principles
Authors: Hsiao-Yi Chen, Takuya Nomoto, Max Hirschberger, and Ryotaro Arita
Journal: Physical Review X