New photoacoustic probes enable deep brain tissue imaging, with the potential to report on neuronal activity and enable better understanding of brain function
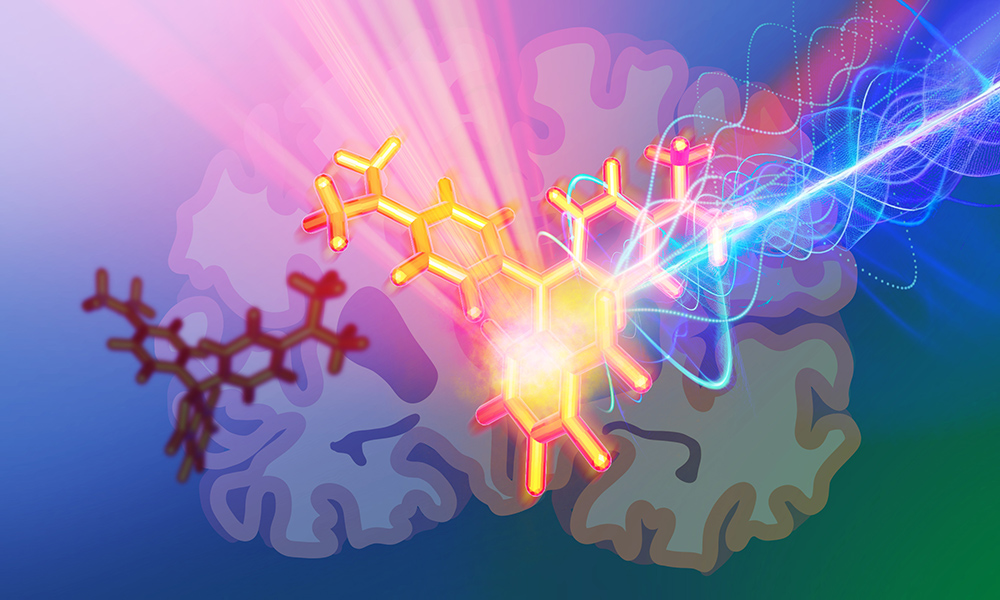
Summary
- Neuroscientists have sought to better understand brain function but lacked the capability to observe neuronal activity deep within the brain.
- EMBL scientists applied rational molecular engineering to develop photoacoustic probes that can be used deep within brain tissue to label and visualise neurons.
- This imaging approach expands significantly on what neuroscientists have been able to see with conventional light microscopy, offering the potential to report on deep neuron activity and better understand brain function.
- This research represents a first proof of principle for a dynamic observation tool that could significantly improve our understanding of brain function.
To understand the brain better, we need new methods to observe its activity.
That is at the heart of a molecular engineering project, spearheaded by two EMBL research groups, that has resulted in a novel approach to create photoacoustic probes for neuroscience applications. The findings were published in the Journal of the American Chemical Society.
"Photoacoustics offer a way to capture imagery of an entire mouse brain, but we just lacked the right probes to visualise a neuron's activity," said Robert Prevedel, an EMBL group leader and a senior author on this paper. To overcome this technological challenge, he worked with Claire Deo, another EMBL group leader and also a senior author on the paper. She and her team specialise in chemical engineering.
"We have been able to show that we can actually label neurons in specific brain areas with probes bright enough to be detected by our customised photoacoustic microscope," Prevedel said.
Scientists can learn more about biological processes by tracking certain chemicals, such as ions or biomolecules. Photoacoustic probes can act as 'reporters' for hard-to-detect chemicals by binding to them specifically. The probes can then absorb light when excited by lasers and emit sound waves that can be detected by specialised imaging equipment. For neuroscience applications, however, researchers have so far been unable to engineer targeted reporters that can visualise brain functions tailored for photoacoustics.
While researchers have experimented with using synthetic dyes as photoacoustic reporters of neuronal activity, controlling where the dye goes and what might be labelled has been challenging. Proteins have been particularly useful as probes for tagging specific molecules, but have not yet led to effective photoacoustic probes to monitor neural activity across the entire brain.
"In our case, we took the best of both of these sensors, combining a protein with a rationally designed synthetic dye, and we can now label and visualise neurons in specific regions of interest," said Alexander Cook, first author of the study and a predoctoral fellow in the Deo group. In rational design approaches, researchers use existing knowledge and principles to build molecules with the desired properties, instead of blindly making and testing random compounds. "Also, we're not just talking about a static observation, but instead this probe shows a reversible, dynamic response to calcium, which is a marker of neuron activity," Cook added.
According to Deo, an important challenge stood in the way of this technological development. Because photoacoustic probes have not been extensively studied, the researchers lacked a way to evaluate the probes they were building.
Consequently, the project began with Nikita Kaydanov, co-author of the study and predoctoral fellow in the Prevedel Group, who custom-made a spectroscopy setup. "There is no commercial setup that can measure photoacoustic signals of a probe in test tubes or cuvettes, so we had to build one," Kaydanov said. "We created our own photoacoustic spectrometer to assess and optimise the probes."
"This allowed us to evaluate and characterise the different probes we made to assess a few things," Deo said. "Did they produce a detectable photoacoustic signal? Are they sensitive enough? That's how we inferred the next steps."
But just producing probes that work in a vial wasn't where the researchers wanted to stop. They then wanted to see how the probes worked in practice. They figured out a way to deliver the probes into a mouse brain and successfully detected photoacoustic signals from neurons inside the targeted brain regions.
"While we are excited about the progress, we need to be clear that this is just the first generation of these probes," Deo said. "While they offer a very promising approach, we have a lot more work to do, but it's a good first demonstration of what this system can enable and the potential it has in better understanding brain function."
In fact, those next steps include improving the dye delivery system and confirming the ability to use them for dynamic imaging inside cells.
"It is really one of the advantages of EMBL that it brings together so many people with different kinds of expertise," Prevedel said. "We're both developers in our own way - my group works more on instrumentation, and Claire's group does more molecular tools. And combining this with neuroscientists who then truly test the tools - this is a special and unique way of doing research, only possible at EMBL."