The fatty membrane surrounding every living cell is a vibrant environment where countless biological processes take place. This membrane protects the cell from its surroundings and divides it into internal compartments; it is responsible for metabolism, energy production, communication with other cells and much more. Most of these processes are performed by proteins embedded within the cellular membrane. In a new study published recently in Nature Communications, researchers from Dr. Nir Fluman 's lab at the Weizmann Institute of Science revealed that thousands of proteins rely on their "tails" to become successfully embedded within the membrane and perform their functions. The researchers further showed that changes to those tails might be responsible for rare genetic disorders in humans.
While some proteins only cross the membrane once, many do so several times, like a thread being woven into a piece of cloth. These proteins are made up of three structural elements: helices embedded inside the membrane, loops connecting these helices, and tails - protein "thread ends" found at the beginning of the first helix and at the end of the last one. The sewing machine-like mechanism that weaves most of the protein helices into the membrane was deciphered decades ago. As part of the embedding process, the ribosome - the cell's protein production machine - attaches to the membrane and, every time a helix is pushed off the production line because the next helix in the chain is being produced, the sewing machine catches it and weaves it into the membrane. Problems can arise, however, in the last stage of the process, when the final helix remains stuck in the pipeline and is inaccessible to the sewing machine. If that helix cannot be successfully woven into the cell's membrane, the whole protein will not function.
In the new study, led by Drs. Ilya Kalinin and Hadas Peled-Zehavi from Fluman's lab in Weizmann's Biomolecular Sciences Department, the researchers sought to uncover the evolutionary solution to the problem of the final helix. It was already known that some proteins have a built-in solution: Their tails are long enough to ensure that, when they are produced, the last helix is pushed off the production line so that it can be successfully woven into the membrane before production of the protein ends. In contrast, many proteins - around 1,400 in the human body - are not so lucky: Their tails are simply too short.
""Membrane protein mutations cause disease, yet most remain a mystery. Understanding their integration could reveal new treatments"
The researchers found that during the evolutionary process these short tails developed hydrophobic properties, meaning that they repel water and are strongly attracted to fatty lipids. This could have helped them cross from the internal side of the fatty membrane to its exterior, thereby lodging the final helix inside the membrane. However, because of the thickness of the fatty membrane, the increase in hydrophobic properties alone is not enough; an additional process is needed to help the tails cross the membrane. To identify this process, the researchers silenced, one by one, every mechanism involved, and they found that disrupting a protein called YidC prevented the final helix from being woven into the membrane.
The discovery of YidC explained why, in the course of evolution, the short tails took on hydrophobic characteristics. Unlike the sewing-machine mechanism, which indiscriminately transports protein sections through the membrane using a channel that ensures their passage, the YidC flattens a certain part of the wide, fatty membrane, thereby helping only sections that are already naturally hydrophobic to cross the membrane more easily. This effectively solves the problem of the final helix.
Armed with these findings, the researchers decided to examine whether problems with short tails could explain some of the rare genetic diseases in humans. Using a database containing genetic sequences of patients' DNA, they identified five genetic diseases caused by mutations that resulted in the loss of hydrophobic properties in the short tail, including one rare genetic defect linked to epilepsy and another connected to an inflammatory condition. The researchers were able to trace the mechanisms of two of the diseases and realized that in both of them, the cells failed to weave in the final helix of the proteins, which caused the proteins to become dysfunctional. Moreover, these proteins were unable to arrive at their proper destination - a cellular organelle in which they are supposed to function - and were found instead inside another organelle that handles faulty proteins.
"Thousands of mutations in membrane proteins lead to human diseases, and we don't understand most of them," Fluman says. "If we can discover which protein sequences are important for getting these proteins into the membrane so that they can function - as we did with clarifying the dynamics of short tails - we can better understand these mysterious genetic diseases and search for effective treatments."
Also participating in the study were Alon Barshap, Shai Tamari, Yarden Weiss and Dr. Reinat Nevo from Weizmann's Biomolecular Sciences Department.
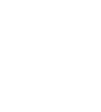
Science Numbers
Proteins that cross the cell's membrane at least 3 times are relatively common in nature: There are around 2,500 such proteins in humans, around 800 in brewer's yeast and approximately 700 in the E. coli bacterium.