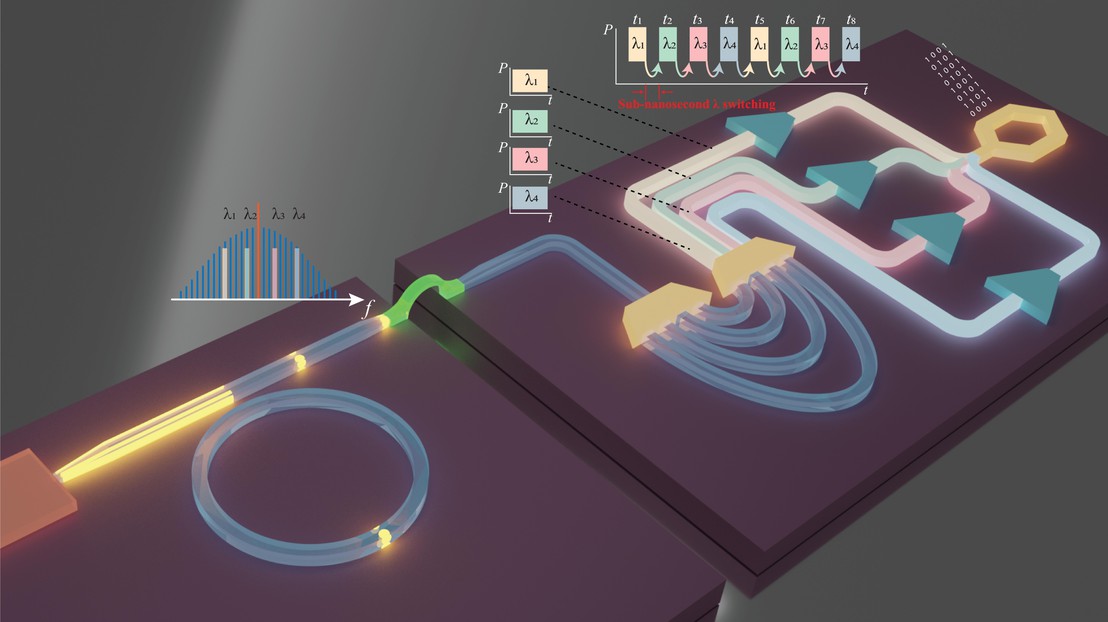
A photonic integrated platform that enables the ultrafast optical circuit switching for datacenters. Credit: Aqeel Ahmed/EPFL
EPFL and Microsoft Research scientists demonstrated ultrafast optical circuit switching using a chip-based soliton comb laser and a completely passive diffraction grating device. This particular architecture could enable an energy-efficient optical datacenter to meet enormous data bandwidth requirements in future.
Services from all hyper-scale cloud providers like Microsoft are powered by massive datacenters that employ hundreds of thousands of servers, whose performance depends heavily on the quality of the network between them. Current datacenter networks include multiple layers of electrical packet switches interconnected through optical fibers. These systems require electrical-to-optical conversion, which increases cost and power overhead. To make things worse, growing data rates due to applications like AI and data analytics could concur with the slowdown of Moore's law which would make it extremely difficult to efficiently scale current network architectures relying on electrical chips.
Optical circuit switches (OCS) are emerging as an exciting option for overcoming bandwidth and scaling issues at datacenters. A particularly promising OCS architecture is wavelength switching where different servers are connected using different colors (wavelengths) of light leading to flatter network architecture and limiting the need for electrical switches and optical transceivers. Switching different wavelengths of light and routing signals to destination servers is done by a switching element, e.g. a glass prism through which different wavelengths can be separated by dispersion.
Although OCS technologies are commercially available today, they are extremely slow, which means they cannot handle increasingly bursty datacenter applications while properly utilizing network resources to reduce overheads and improve power consumption.
In a new paper published in Nature Communications, research teams led by Professor Tobias J. Kippenberg at EPFL and by Dr Hitesh Ballani at Microsoft Research Cambridge have successfully demonstrated ultrafast OCS for datacenters by using chip-based optics. The research teams have collaborated since 2018 as part of the Microsoft Swiss Joint Research Center.
In the proposed architecture, optical microcombs act as a multiwavelength source providing coherent carriers. Optical amplifiers and arrayed waveguide gratings based on semiconductor materials perform the switching and separate or combine the different colors of light respectively.
Optical microcombs, pioneered by Kippenberg's group, provide hundreds of equidistantly spaced carriers that are suitable for many applications. The microcomb sources are generated through nonlinear frequency-conversion by using a chip-scale silicon nitride microresonator, presenting unique advantages in power and size over the laser arrays conventionally used as multi-wavelength sources.
Silicon nitride microresonators are fabricated using the photonic damascene process, a CMOS-compatible technique that features ultra-low propagation loss, which is extremely critical to make power-efficient microcomb sources.
The chip-scale indium phosphide-based optical amplifiers, fabricated using commercial foundries, perform the switching between different colors of light at sub-nanosecond timescales. This ultra-fast switching between different microcomb carriers is important for meeting the performance requirements of modern and future datacenter applications.
A proof-of-concept, system-level demonstration showed that data transmission with packet-by-packet switching can be achieved and hence, has the potential to meet the requirements of datacenter applications. Finally, the researchers present a unique architecture that employs a central comb system to improve power efficiency and reduce complexity.
"Soliton microcombs have been used in many key system-level applications such as LiDAR, long-haul data transmission, and optical coherence tomography since their discovery back in 2014," says Kippenberg. "The potential use of microcombs in datacenters to meet future bandwidth requirements and reduce power consumption further consolidate the importance of this platform for scientific and technological applications."
The silicon nitride samples were fabricated and grown in the Center of MicroNanoTechnology (CMi) at EPFL.