Researchers in the Galej Group at EMBL Grenoble have provided new insights into the structure of the minor spliceosome, an essential RNA-protein complex
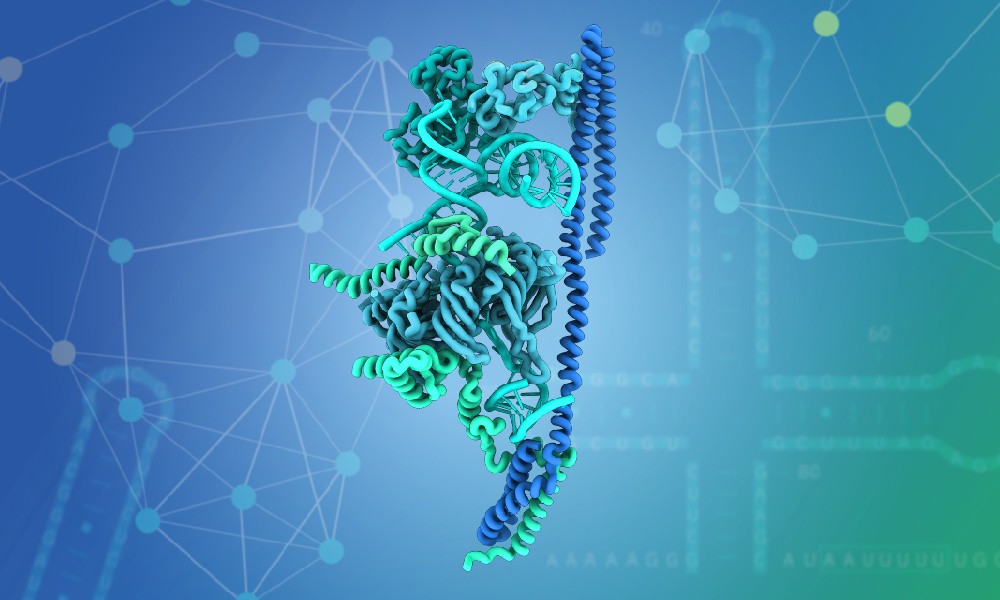
Summary
- Human genes that encode proteins often contain non-coding segments known as introns. Removing introns is crucial for the proper expression of genetic information.
- Understanding how our cells differentiate between coding and non-coding genetic material is crucial to human health.
- A specialised molecular complex known as the minor spliceosome removes some rare but critical introns, and several genetic disorders are associated with its dysfunction.
- The Galej Group at EMBL Grenoble has provided new insights into minor spliceosome function, revealing the structure of U11 snRNP - one of its subunits, which initiates the intron selection process.
In human cells, only a small proportion of the information written in genes is used to produce proteins. How does the cell select this information? A large molecular machine called the spliceosome constinuously separates the coding and non-coding regions of our genes - and it's doing this even as you read these lines.
The spliceosome is critical for the proper functioning of every cell, and numerous genetic disorders are linked to problems with spliceosome function. In most eukaryotic cells, two types of spliceosome work in parallel to stitch gene pieces together: the major spliceosome and the minor spliceosome.
The Galej Group at EMBL Grenoble has recently published new structural insights into minor spliceosome function in the journal Molecular Cell .
The major spliceosome is abundant in cells and has been extensively investigated for more than four decades. Its long-separated twin - the minor spliceosome, is much more scarce and remains enigmatic, even though its function is equally important. In this new study, the researchers reveal the structure of U11 snRNP - one of the five subunits of the minor spliceosome, which initiates the intron selection process.
Stitching gene pieces together
Spliceosomes help cells remove large chunks of non-coding genetic information - called introns - from the precursors of messenger RNA (pre-mRNA), the molecule responsible for transferring genetic information from DNA into proteins. Spliceosomes act as an editing tool, helping to make sense of the fragmented messages stored in pre-mRNA.
Most genes have many introns that belong to one class - the major introns - which are removed by the major spliceosome. However, around 0.5% of introns belong to another class - the minor introns - which are processed by the minor spliceosome.
"These minor introns are very rare but extremely critical because they are often located in housekeeping genes that are essential for supporting life," said Jiangfeng Zhao, a postdoctoral fellow in the Galej Group and the first author of the study.
Spliceosomes are large and dynamic RNA-protein complexes. The major spliceosome consists of five subunits, U1, U2, U4, U6, and U5 small nuclear ribonucleoprotein particles (snRNPs, read "snurps") - and about 150 proteins, involved in different stages of the splicing process. The minor spliceosome has a similar architecture, but its main building blocks are different (U11, U12, U4atac, U6atac, and U5 snRNPs). How exactly these blocks assemble to build the minor spliceosome and help it fulfil its function remains elusive.
Structures of large molecular machines are notoriously difficult to determine because of their intrinsic dynamic nature. This is even more true for molecular machines that are scarce in cells, like the minor spliceosome. "One of the biggest challenges in studying this complex was to figure out how to selectively purify it from entire cell content," added Zhao. "Once we managed that, it still took several years of optimisation to keep the complex intact under imaging conditions."
Long-separated twins: at the crossroads of evolution
EMBL Grenoble Group Leader Wojciech Galej, who has been working on pre-mRNA splicing machinery for more than 15 years, first decided to focus on the minor spliceosome seven years ago - a project for which he obtained an ERC Starting Grant in 2020.
When the Galej Group started the project, little was known about the minor spliceosome, and even now, only a handful of research groups are doing structural studies on this RNA-protein complex.
"The major and minor spliceosomes are evolutionarily related, but, as far as we know, the last common ancestor of all eukaryotes most likely already had both types of spliceosomes," said Wojciech Galej, the corresponding author of the study. "This means that they diverged more than 1.5 billion years ago, a timescale very difficult to imagine."
Using biochemistry and cryo-electron microscopy, the researchers obtained the structure of the U11 snRNP complex and revealed how it recognises the "5' splice site" - the starting point of an intron - to initiate the editing process at this precise location.
The results show that the architecture of U11 snRNP is strikingly different from that of U1 snRNP - the corresponding subunit of the major spliceosome. "The different and more complex architecture allows the U11 snRNP to specifically identify its rare substrates in the vast RNA sequence landscape of every cell," explained Zhao. "It is like finding a needle in a haystack. Minor spliceosome has evolved to use additional non-canonical base-pairing interactions to fulfil this goal with high fidelity."
Defining where to start cutting out the introns is just the first step in the spliceosome's complex assembly pathway. At least a dozen other steps are needed before the splicing reaction concludes. Not much is known about how the minor spliceosome transitions through these intermediate steps.
"This has been a challenging project, and we're making progress along the way, which is encouraging, but there's still so much to know about the minor spliceosome," said Zhao, who was awarded a Marie Skłodowska-Curie grant in 2023 to study the other stages of minor spliceosome activity.
"Our work provides exciting new insights into the mechanism of minor intron recognition and sheds light on the evolution of the splicing machinery. It opens up new possibilities for studying other minor spliceosome complexes," said Galej. "Our long-term goal is to understand how this entire pathway works at the molecular level, and we hope that this and future work will contribute to a better understanding of the molecular basis of the genetic disorders associated with the components of the minor spliceosome. This could ultimately lead to new therapeutic applications."