Two experiments have come to wildly different results. Now what? A mass mystery gets a cross check.
DURHAM, N.C. -- One of the tiniest building blocks of the universe has a weigh-in problem, and Ashutosh Kotwal is determined to get to the bottom of it.
For nearly 30 years, the Duke physicist has led a worldwide effort to hone in on the mass of a fundamental particle called the W boson .
It's the force-carrying particle that allows the Sun to burn and new elements to form, so it's pretty important. Without it, the entire universe would be in the dark.
But in recent years the W boson has been the source of a rift in the physics world. That's because the two most precise measurements to date of its mass -- essentially how much matter, or "stuff" the particle contains -- don't line up.
What's at stake is more than a subatomic version of a carnival weight guessing game. The question is whether our understanding of the laws of nature needs to be rewritten.
And now, in the first of a series of new studies, Kotwal examines what might be behind this measurement conundrum -- and how to solve it.
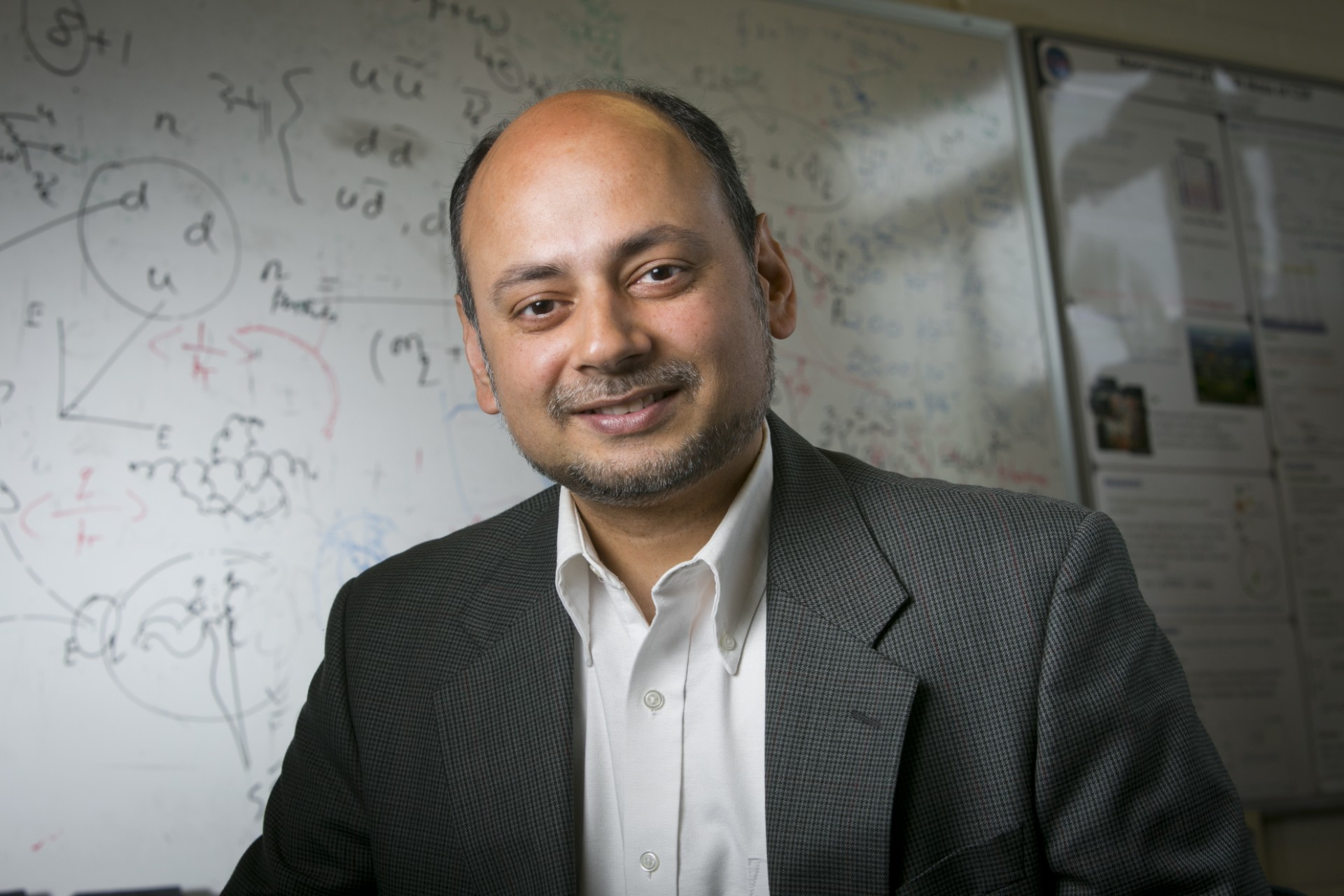
Oversized or not?
It all started a few years ago, Kotwal said, when he and a team of physicists sifting through data from the Fermi National Accelerator Laboratory near Chicago got a curious surprise.
Their analysis of the CDF experiment , based on some four million W bosons produced by what was once the world's most powerful particle accelerator, suggested that the particles were significantly heftier than predicted by the most important theory in particle physics.
Physicists measure the mass of subatomic particles in units called electron volts, or eV. According to theory, the W boson ought to have a mass very close to 80,357 million eV, or about 80 times the mass of a proton.
But the team's measurement -- which took 10 years to make and was the most precise mass measurement ever made for the W boson -- came in at a whopping 77 million eV heftier, and with only a 0.01% margin of error. It was too big of a discrepancy to be written off as a fluke.
The findings raised the possibility of cracks in the Standard Model of particle physics, our best explanation of how particles combine and interact to create the world around us.
But a subsequent measurement, released last year by researchers at CERN and almost as precise, cast the W boson in a more humdrum light.
The result, the first W mass measurement from the CMS experiment at CERN's Large Hadron Collider in Switzerland, failed to find anything unusual or out of the ordinary. Rather, that team had closed in on exactly the mass predicted by physicists' standard model.
In other words, just like that -- poof -- the anomaly was gone.
The mismatch between the two experiments suggests that one or both teams has missed something.
"We can't both be right," Kotwal said.
It's possible, he said, that there is "a potential flaw hiding in one of our techniques," or that one of the teams overlooked some source of error.
Since then, Kotwal has been working to understand the roots of the discrepancy, picking apart every aspect of their analyses in search of an explanation for why the two groups arrived at such different answers.
How to weigh a boson
Part of the trouble is that it's a fiendishly tricky measurement to make.
Since the W boson was discovered in 1983, teams of scientists using different detectors have made multiple attempts to measure its mass. But they can't just put it on a scale and weigh it.
For one, it's extremely short-lived. W bosons are made by smashing beams of particles together at nearly the speed of light inside powerful particle colliders like the Tevatron at Fermilab.
But the W bosons spit out by these collisions break apart almost as soon as they are formed, showing up in particle colliders for less than a trillionth of a trillionth of a second before they decay.
So, scientists have to weigh the W boson indirectly, inferring its mass by studying the showers of particles the decaying bosons produce.
To get the mass measurement right, Kotwal said, researchers must first carefully measure the energies and momenta of these flying debris particles as they are glimpsed by the detector.
By measuring their kinetic energy -- essentially how much "oomph" they have as they fly out -- the researchers are able to work backwards, using the laws of energy and momentum conservation, to determine the mass of the W boson.
In a new study published in Physical Review Research, Kotwal puts those calculations to the test.
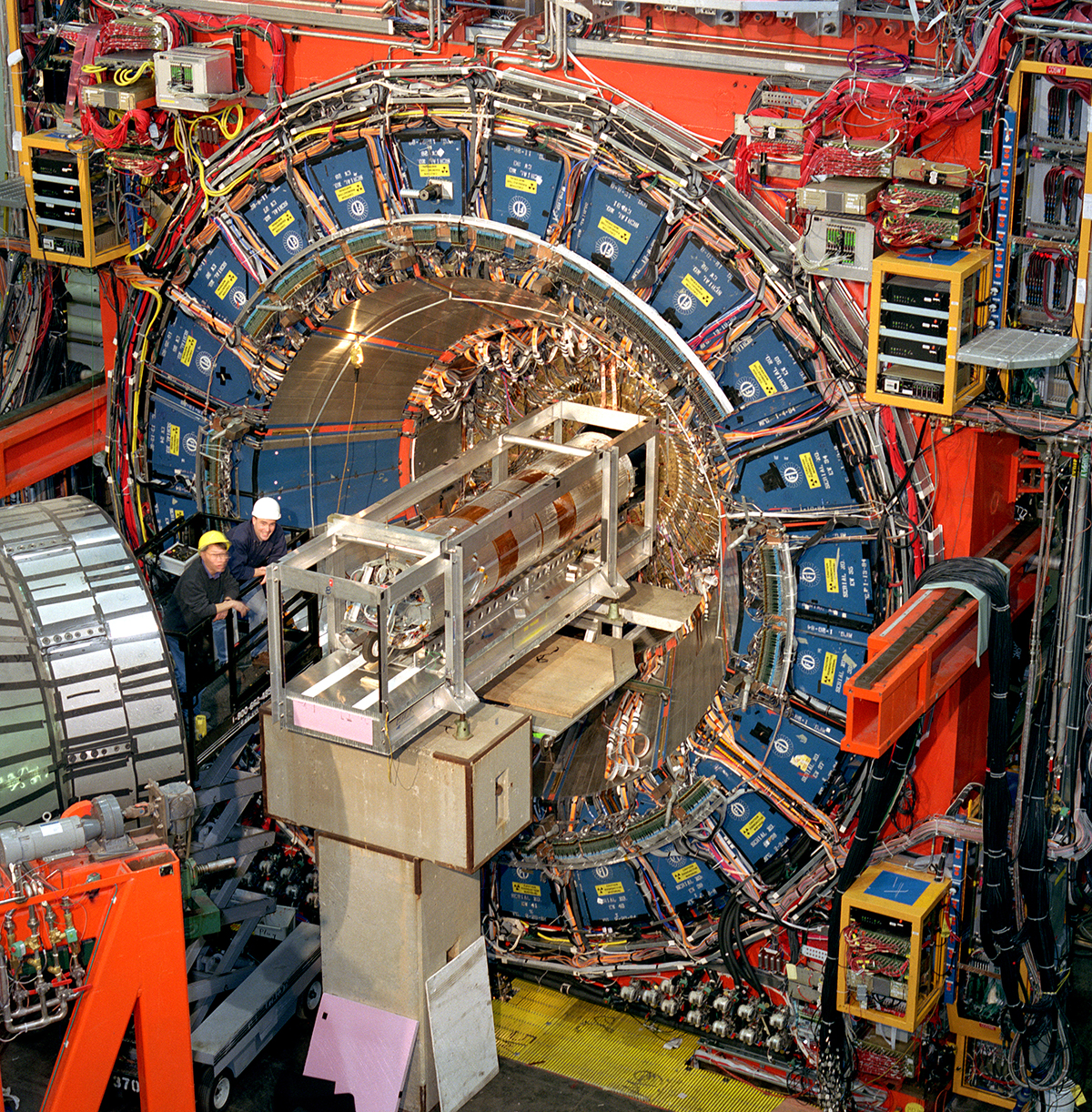
Flight paths
The mass measurement from the Fermilab team is based on an analysis of 4.2 million W bosons produced at a particle collider called the Tevatron between 2002 and 2011.
The key part of their experiment is a can-shaped chamber packed with 30,240 high voltage wires that record and determine the 3D position of each decay particle at 96 different points as it whizzes outward from the point of collision.
But the particles don't move in straight lines. They trace an arc as they curve around the chamber's magnetic field in a predictable path.
By essentially drawing a curve through the series of signals they leave behind, the CDF scientists are able to very precisely determine each particle's momentum and other properties.
"It's a lot like the connect-the-dots game we used to play as children," Kotwal said.
Kotwal says the team spent four years ensuring they could reconstruct each particle's trajectory with exquisite precision, using sophisticated algorithms to pinpoint the position of each of the tens of thousands of wires to within a millionth of a meter.
It's still possible, Kotwal said, that some error in tracing this flight path could be throwing them off.
"What if the wires were not exactly where we thought they were, and we didn't realize it?" Kotwal said. "Suppose the wire chamber was squeezed or bent in a particular way, then all the wire positions would be slightly off."
So, Kotwal examined all the possible subtle wire misalignments that could put their result in doubt.
In the first results of an internal audit published this month, he reported the findings.
"All possible shifts were checked and found to be too small to matter," Kotwal said. Even taking miniscule possible shifts in the wires within the chamber into account, a particle's kinetic energy can still be measured to the quoted accuracy of 25 parts per million.
"From this perspective, the CDF measurement checks out", Kotwal said.
There's more work to be done to sort out the reason for the gulf between the two experiments, he added.
But the goal is to pull back the curtain so that every step is laid bare and other researchers can see how they arrived at their answer.
"When you go after a fundamental parameter of nature, the methods cannot be opaque. They need to be fully transparent," Kotwal said.
Kotwal says he hopes other experiments will do the same.
Figuring out what we're getting wrong is the key to getting it right, he added.
"What if we overlooked something here? It's our duty to go check," he said, stressing that it's too early to declare the case closed for hopes for new physics.
"The thing that we all ought to be doing is not jumping to some conclusion, but to dig in and figure out which methods are right and which methods are wrong."
"That's how scientists should do it, right?"
Citation
"Model for the Curvature Response of the CDF II Drift Chamber," Ashutosh V. Kotwal. Physical Review Research, Feb. 3, 2025. DOI: 10.1103/PhysRevResearch.7.013128